- Abstract:
- 1 Introduction▲
- 2 Materials and methods▲
- 3 Results and discussion▲
- 4 Conclusions▲
- References
- Figure
- Fig.1 Phylogenetic tree for JDC-16 showing relationships between strain and selected close members of genus Acinetobacter (Numbers at branch nodes are bootstrap values based on 1 000 re-samplings, and nucleotide sequence accession number for each reference strain is indicated in brackets)
- Fig.2 Effect of pH on microbial biomass (OD600) and DEP degradation by Acinetobacter sp. JDC-16 at 30 ℃ and initial DEP concentration of 500 mg/L
- Fig.3 Effect of temperature on microbial biomass (OD600) and DEP degradation by Acinetobacter sp. JDC-16 at pH 7.0 and initial DEP concentration of 500 mg/L
- Fig.4 Best-fitted curves for DEP degradation using modified Gompertz mode at different initial concentrations by Acinetobacter sp. JDC-16
- Fig.5 Microbial biomass and DEP degradation by DEP-induced and non-induced bacterial cells of Acinetobacter sp. JDC-16: 1—DEP degradation with induction; 2—DEP degradation without induction; 3—OD600 with induction; 4—OD600 without induction
J. Cent. South Univ. Technol. (2010) 17: 959-966
DOI: 10.1007/s11771-010-0584-3
Aerobic biodegradation of diethyl phthalate by Acinetobacter sp. JDC-16 isolated from river sludge
LIANG Ren-xing(梁任星), WU Xue-ling(吴学玲), WANG Xing-na(王兴娜),
DAI Qin-yun(代沁芸), WANG Yang-yang(王洋洋)
School of Minerals Processing and Bioengineering, Central South University, Changsha 410083, China
? Central South University Press and Springer-Verlag Berlin Heidelberg 2010
Abstract:
A gram negative bacterium, named JDC-16, which can grow well on the substrate of phthalic acid esters (PAEs) as the sole source of carbon and energy, was isolated from river sludge. Based on the morphology, physiological and biochemical properties and analysis of 16S rRNA gene sequence, it was preliminarily identified belonging to the genus Acinetobacter. The result of substrates utilization range indicates that strain JDC-16 can utilize a variety of phthalates except for diisononyl phthalate (DINP). The degradation tests using diethyl phthalate (DEP) as the model compound show that the optimal pH and temperature for DEP degradation by Acinetobacter sp. JDC-16 is 8.0 and 35 ℃, respectively. Meanwhile, degradation kinetics under various initial concentrations of DEP reveals that substrate depletion curves fit well with the modified Gompertz model with high correlation coefficient (R2>0.99). Furthermore, the substrate induction test indicates that DEP-induction can apparently shorten the lag phase and enhance the degradation rate. This work highlights the potential of this isolate for bioremediation of phthalates-contaminated environments.
Key words:
biodegradation; diethyl phthalate; kinetics; Acinetobacter sp.; river sludge;
1 Introduction
Phthalic acid esters (PAEs) are a group of chemicals widely used in the manufacture of plastics and many other industrial and consumer products [1]. Diethyl phthalate (DEP) is one of the important molecules in the family of PAEs that are typically used in cellulose ester-based plastics, such as cellulose acetate and butyrate. During the last decades, DEP has been found to have diverse acute and chronic toxic effects on several species at different trophic levels, as well as endocrine-disrupting properties [2]. In addition, PAEs including DEP have been found to be potentially harmful for human because of their hepatotoxic, teratogenic, and carcinogenic characteristics [3]. Therefore, DEP and other phthalates have been listed as priority pollutants and endocrine-disrupting chemicals (EDCs) by the China National Environmental Monitoring Center [4] and the US Environmental Protection Agency [5].
Because DEP is not chemically bound to the products to which they are added, it can easily migrate into the environment. So far, DEP has been detected in landfill leachate, surface water, sewage sludge, sediment, soils and even atmosphere [6]. Hence, it is critically important to remove phthalates from the environment effectively and economically. The degradation of phthalates in environment can occur by hydrolysis, photolysis and biodegradation. Numerous studies demonstrated that metabolic breakdown of PAEs by microorganisms was one of the major routes of environmental degradation for these widespread pollutants due to the low rate of chemical hydrolysis and photolysis in the environment [1].
To date, the biodegradation of DEP under various environment conditions such as wastewater treatment processes [7], sludge [8], soils [9] and river sediment [10] has been widely investigated. The average half-life of DEP in river sediments was reported to be 2.5 d in aerobic environment and 33.6 d in anaerobic environment [8]. In addition, several bacterial strains belonging to the genera Sphigomonas, Corynebacterium, Pseudomonas and Acinetobacter were isolated from various environments for the biodegradation of DEP [11-14]. So far, there has been only one report of the species in the genus Acinetobacter capable of degrading DEP. Moreover, most of the characteristics of these bacteria remain unclear.
In this work, an aerobic DEP-degrading bacterial strain isolated from river sludge was characterized.
The effects of pH and temperature on the biomass and DEP degradation were investigated to determine the optimal degradation conditions. In addition, the kinetics of DEP biodegradation under various initial concentrations was also studied to determine relevant kinetic parameters under aerobic conditions.
2 Materials and methods
2.1 Chemicals
Di-n-octyl phthalate (DOP) and diisononyl phthalate (DINP) were purchased from Aldrich-Sigma Chemicals (St. Louis, Missouri, USA) with 99% purity. Dimethyl phthalate (DMP), diethyl phthalate (DEP), di-n-butyl phthalate (DBP), diisooctyl phthalate (DIOP), all with 99.5% purity, were obtained from China National Medicine Group. All other chemical reagents were of analytical grade and solvents were of HPLC grade.
2.2 Source of microorganisms
The bacterium used in the degradation experiment was isolated from river sludge collected from Tangshan, Hebei Province, China. The sludge samples were stored in plastic bags and kept at 4 ℃ before being used.
2.3 Enrichment and isolation of bacteria
The minimum salt medium (MSM), used in all cases including enrichment experiments unless otherwise stated, contained the following chemicals in 1 L of distilled water: K2HPO4 5.8 g, KH2PO4 4.5 g, (NH4)2SO4 2.0 g, MgCl2 0.16 g, CaCl2 0.02 g, Na2MoO4?2H2O 0.002 4 g, FeCl3 0.001 8 g, MnCl2?2H2O 0.001 5 g. The pH of MSM was adjusted with HCl or NaOH to 7.0 and then sterilized by autoclaving at 121 ℃ for 20 min. The solid plates were prepared by adding 18 g of agar to 1 L of the liquid medium.
The enrichment procedure was similar to that described by CHAO et al [15] with some modifications. Initially, 5.0 g of sludge was added to a 500-mL Erlenmeyer flask containing 200 mL of MSM solution amended with a mixture of PAEs (total, 200 mg/L; DMP, DEP, DBP and DOP, each 50 mg/L). The suspension was incubated in the dark at 30 ℃ for 7 d on a rotary shaker operated at 170 r/min. Subsequently, 2 mL of the enrichment culture was continuously transferred four times to fresh medium (each time containing a higher concentration of PAEs (from 240 to 500 mg/L)) and incubated under the same conditions. Then, the final enrichment was streaked onto MSM agar (18 g/L) plates supplemented with a mixture of PAEs (500 mg/L) and incubated at 30 ℃ for 7 d. Presumptive colonies were picked based on differences in colony morphology and coloration and re-streaked onto MSM agar plates amended with the mixture of PAEs. The bacterial isolates were further purified by streaking on Luria-Bertani (LB) nutrient agar plates and then re-streaked onto MSM agar plates with and without PAEs to confirm their degradation abilities. Isolates showing growth in the presence of PAEs (but not in their absence) were selected for further study. The pure cultures obtained were stored in 0.05 mol/L potassium phosphate buffer (pH 7.5) containing 30% (volume fraction) glycerol at -70 ℃.
2.4 Identification and characterization of bacterial strain
Gram reaction and cell morphology were determined by observing stained cells under a light microscope. Biochemical tests for the identification of bacteria were performed using standard methods [16]. The bacterial isolates of pure cultures were further characterized using 16S rRNA gene sequencing methods. To extract the genomic DNA of bacterial strains for 16S rRNA gene amplification, 2 mL of bacterial culture grown in liquid MSM amended with PAEs was collected and centrifuged. The cell pellet was washed three times with phosphate–buffer saline (PBS, 0.05 mol/L, pH 7.4) and then re-suspended in 200 ?L of Tris-HCl/EDTA (TE) buffer (pH 8.0). Genomic DNA was extracted using an EZ-10 Spin Column Genomic DNA Minipreps Kit (Bio Basic Inc., Markham Ontario, Canada). Then, the 16S rRNA gene from genomic DNA was amplified with bacterial universal primers F27 and R1492 [17]. The PCR products were purified using an E.Z.N.A? Gel Extraction Kit (Omega Bio-Tek, Inc., USA) and then sequenced (Sangon, Shanghai, China). The 16S rRNA gene sequence of the bacteria were aligned and compared with the bacterial gene sequences in the GenBank. The closest references were retrieved from NCBI GenBank and aligned with CLUSTALW with all parameters set at their default values. Phylogenetic tree was then constructed using the neighbor-joining method with MEGA 4.1 [18].
2.5 Substrates range tests
Liquid MSM was supplemented with one of the following substrates, which were protocatechuate (PCA), phthalic acid (PA), isophthalic acid (IA), terephthalic acid (TA), DMP, DEP, DBP, DOP, DIOP and DINP (200 mg/L), as carbon sources to examine the ability of PAEs-degrading bacterial strains to utilize these compounds. Among them, PA and PCA were dissolved in chloroform and extracted through 0.22 μm membrane. The others were added into the MSM medium directly after being autoclaved. Growth was followed by measuring the increase of optical density of biomass production at 600 nm (OD600) and the light microscope observation.
2.6 Effects of pH and temperature
The effects of pH and temperature on microbial biomass and degradation were investigated to determine the optimal degradation conditions. Strain JDC-16, grown in the MSM supplemented with PAEs at 30 ℃ on a rotary shaker operated at 150 r/min, was harvested after 36 h. Then, the harvested cells were washed with 0.05 mol/L potassium phosphate buffer (pH 7.5) three times under sterile conditions and re-suspended in the same phosphate buffer, resulting in a cell suspension with OD600 of 0.2. The degradation of DEP (500 mg/L) was conducted in 100 mL of sterilized MSM inoculated with 1 mL of the prepared cell suspension in 250 mL Erlenmeyer flasks. The initial pH was adjusted to 4.0, 5.0, 6.0, 7.0, 8.0, 9.0, and 10.0 by adding 1 mol/L NaOH or HCl solution and the batch cultures were incubated at 30 ℃ on a rotary shaker operated at 150 r/min in the dark. Under the same shaking condition, the incubation temperatures were set at 20, 25, 30, 35, 40 and 45 ℃, respectively under condition of pH 7.0.
2.7 Effect of DEP concentration
Afterward, the degradation kinetics was investigated under various initial concentrations of DEP. Experiments on DEP degradation were carried out at the identified growth pH and temperature in 250 mL shaking flasks. Each flask contained 100 mL of fresh feed solution with DEP at concentrations of 50, 100, 150, 200, 300 and 500 mg/L. The medium was then inoculated and incubated in the same way as described previously. Mixed liquor samples were sampled every 3 h for DEP and biomass concentration analyses.
2.8 Substrate induction test
To prepare the DEP-induced cells, the bacterial isolate was cultured in MSM containing DEP (500 mg/L) to mid-log phase, and, in contrast, the DEP non-induced cells were cultured in LB medium to mid-log phase. The cell suspension was prepared as described previously. The cell suspension (1.0 mL) was inoculated into a fresh MSM containing DEP (200 mg/L) to determine degradation capability. All the flasks were incubated under the identified optimal conditions. Periodically, cultures (2 mL) were sampled every 3 h and 1 mL was preserved at -20 ℃ for further high performance liquid chromatography (HPLC) analysis and the other 1 mL was used for OD measurement.
2.9 Analytic methods
The samples were thawed and filtered by passing through 0.2 μm syringe filter prior to HPLC analysis. Quantification of DEP was carried out by HPLC (Elite series; Elite, Dalian, China) equipped with a UV230+ UV-Vis detector and a Hypersil BDS-C18 (200 mm× 4.6 mm, 5 μm) chromatography column. The mobile phase contained methanol/water (60:40, volume ratio) at a flow rate of 1 mL/min. Aliquots of 20 μL culture medium were injected and run at 35 ℃. The UV absorption spectrum of DEP was measured at 280 nm. The quantification was accomplished by external standard (concentration ranging from 12.5 to 500 mg/L, R2=0.997 5).
Meanwhile, the microbial biomass in culture flasks was determined by optical density measurements at 600 nm (OD600) using a 725N UV-Vis spectrophotometer. All the degradation experiments were performed in triplicates with the control without bacteria.
3 Results and discussion
3.1 Identification and characterization of isolates
After enrichment culturing, a bacterial strain (JDC-16) is obtained from enrichment culture inoculated with river sludge using mixtures of PAEs as the sole source of carbon and energy. It is found to be a gram-negative, nonsporulating, and aerobic rod-shaped bacterium. The colonies formed on LB agar medium are yellowish, 1-3 mm in diameter, convex and circular. Physiological and biochemical characteristics of strain JDC-16 are listed in Table 1. By comparison, JDC-16 is similar to other bacteria of the genus Acinetobacter [16]. Based on the biochemical tests, strain is further characterized using 16S rRNA gene sequencing methods. According to BLAST analysis of its 16S rRNA gene sequence, strain JDC-16 seems to be a species in the genus Acinetobacter. Fig.1 illustrates the phylogenetic relationship of JDC-16 with species of close similarity. As shown in Fig.1, strain JDC-16 is closely clustered with Acinetobacter genomosp 13TU strain LUH 3144 with 100% similarity. The accession number of JDC-16 in GenBank is FJ975124.
Although the degradation of DEP by microorganisms from different environments has been reported, there is only one report on DEP degradation by pure culture of Acinetobacter [11]. Besides, another strain Acinetobacter lwoffii ISP4 was reported to be isophthalate rapidly [19]. Actually, Acinetobacter species are widespread in nature and can be obtained from various sources such as river water [11], soil [19], activated sludge [20], granules [21] and effluent treatment
Table 1 Physiological biochemical characteristics of JDC-16
Fig.1 Phylogenetic tree for JDC-16 showing relationships between strain and selected close members of genus Acinetobacter (Numbers at branch nodes are bootstrap values based on 1 000 re-samplings, and nucleotide sequence accession number for each reference strain is indicated in brackets)
plants [22]. Most of the Acinetobacter species are found to be capable of degrading a variety of pollutants such as phenol, 4-chlorophenol and atrazine. The Acinetobacter species have highly hydrophobic cell surface [23] and thus may play a significant role on the biodegradation of various pollutants in the environment.
3.2 Substrates utilization range
The results of substrates tests indicate that there are differences in terms of the types of PAEs and phthalate isomers (Table 2), revealing that JDC-16 shows better growth in the culture medium amended with shorter alkyl-chains phthalate esters such as DMP, DEP and DBP than in that with longer alkyl-chains like DOP, DIOP and DINP. This may lie in that the side chain of the substrates has a significant effect on the biodegradation of PAEs. In fact, previous studies prove that phthalates with shorter ester chains can be more readily biodegraded and mineralized than those with longer ester chains [12-13]. According to these previous studies, the biodegradability difference of phthalates is likely due to the steric effect of phthalates side ester chains, which hinders the hydrolytic enzymes from binding to the phthalates and thereby inhibits their hydrolysis [1]. In addition, JDC-16 can grow well with PA as the sole source of carbon and energy but cannot utilize its isomers isophthalate and terephthalate (Table 2). In 2006, VAMSEE-KRISHNA et al [19] isolated three strains of Pseudomonas aeruginosa PP4, Pseudomonas sp. PPD and Acinetobacter lwoffii ISP4 capable of utilizing phthalate isomers with enrichment culture technique. Among them, strains PPD and PP4 can utilize all three phthalate isomers (PA, TA and IA) as the sole carbon source, whereas ISP4 can only utilize isophthalic acid (IA). These results suggest that the esterases responsible for hydrolysis of the phthalate isomers are highly substrate-specific due to their different structures and conformations [24].
Table 2 Growth profile of JDC-16 on various PAEs and phthalate isomers
3.3 Optimum DEP degradation conditions by Acinetobacter sp. JDC-16
The optimal pH and temperature for DEP (500 mg/L) degradation by Acinetobacter sp. JDC-16 were examined. As shown in Fig.2, strain JDC-16 grows well at pH 7.0-9.0 and shows the best growth at pH 8.0, 30 ℃ and an agitation speed of 150 r/min. Meanwhile, the degradation rate is relatively high corresponding to the big microbial biomass and the highest degradation rate (98.74%) is found at pH 8.0. Interestingly, about 52.16% of DEP is transformed even when pH increases to 10.0. This indicates that the strain can survive under slight alkalic condition. However, JDC-16 can hardly grow at pH 11.0 or 4.0 and 5.0. At the same time, the concentration of DEP is not significantly decreased.
Fig.2 Effect of pH on microbial biomass (OD600) and DEP degradation by Acinetobacter sp. JDC-16 at 30 ℃ and initial DEP concentration of 500 mg/L
Bacterial growth is sensitive to environmental temperature. As shown in Fig.3, the DEP degradation is dependent on temperature. It is observed that the degradation rate of DEP by Acinetobacter sp. JDC-16 increases gradually with increasing temperature between 20 and 35 ℃. The highest degradation rate is achieved
Fig.3 Effect of temperature on microbial biomass (OD600) and DEP degradation by Acinetobacter sp. JDC-16 at pH 7.0 and initial DEP concentration of 500 mg/L
when the temperature sustains between 30 and 35 ℃. Further increase in temperature (>35℃) results in a remarkable decrease in both the degradation rate and microbial growth. No significant decrease of DEP is observed when JDC-16 is incubated at higher (45 ℃) and lower (20 ℃) temperatures. The highest degradation rate (98.38%) and maximum microbial biomass (0.582) are observed at 35 ℃, at which the degradation related enzyme reaches the highest activity. In a previous study, it is reported that the condition for the biodegradation of DEP by strain DEP-AD1 is 25 ℃ and pH 6.0 [14]. However, the optimal conditions for the degradation of DEP by Acinetobacter sp. JDC-16 are found to be 35 ℃ and pH 8.0. These results indicate that strain JDC-16 may be of great potential in degrading DEP in slight alkalic and relative high temperature environment.
3.4 Kinetic analysis of DEP degradation
The kinetics of DEP degradation by Acinetobacter sp.JDC-16 was investigated at different initial concentrations ranging from 50 to 500 mg/L. Results in Fig.4 show that the degradation of DEP follows the modified Gompertz model [24-25], which can be expressed as
(1)
where S is the substrate concentration; S0 the initial substrate concentration; Rm the maximum substrate transformation rate; t0 the lag phase time; and t the incubation time. As shown in Fig.4, the DEP depletion curves are described well by the modified Gompertz model with high correlation coefficient (R2>0.99). According to Fig.4, 50 mg/L of DEP is completely degraded after 15 h of incubation, whereas it takes 27 h
Fig.4 Best-fitted curves for DEP degradation using modified Gompertz mode at different initial concentrations by Acinetobacter sp. JDC-16
to completely remove the DEP when the initial DEP concentration is increased to 500 mg/L. The degradation rate of DEP by Acinetobacter sp. JDC-16 is much higher than those previous reports on DEP degradation by pure cultures [11-14]. The calculated parameters and their R2 are listed in Table 3, which reveal that the degradation of DEP requires a relative short lag phase and increases with the increase of DEP concentrations, from as short as 4.390 6 h to as long as 6.931 2 h. Then, DEP is rapidly degraded after the lag phrase and the maximum transformation rate (Rm) also increases with the increase of DEP concentration. According to these results, the degradation rate is concentration-dependent and the initial concentration of DEP may play an important role in the degradability of DEP.
Table 3 Kinetic parameters of aerobic DEP degradation by Acinetobacter sp. JDC-16 at 35 ℃ and pH 8.0
Several models including the first-order kinetic model, modified GOMPERTZ model and RICHARDS model were used to describe the degradation kinetics of pollutants [25-26]. Among them, the first-order kinetic model was used frequently to describe the biodegradation of xenobiotics at low substrate concentrations. For example, XU et al [27] reported that the biodegradation of DBP at very low concentrations of 2.5-10.0 mg/L could be well described using the first-order kinetic model. In addition, the degradation of DEP at low concentrations of 5-100 mg/L also followed the first- order kinetic model by a strain of Sphingomonas [12]. On the other hand, the degradation of DEP at moderate concentrations of 25-400 mg/L by Pseudomonas fluoresences FS1 isolated from river sludge also followed the first-order kinetic model [13]. Recently, the modified Gompertz model has been widely used to describe the degradation kinetics of PAEs. For example, LI et al [24] reported that the kinetics of DMT, DMI and DMP transformation was well described by the modified Gompertz model. And in 2007, HERBERT et al [14] reported that the degradation of DEP also followed the modified Gompertz model with initial concentrations varying from 39 to 610 mg/L. In addition, another report showed that kinetics of DMP degradation by Burkholderia cepacia DA2 fitted the modified Gompertz model well with various initial concentrations ranging from 200 to 800 mg/L [28]. In this work, it was found that DEP depletion curves by JDC-16 also fitted well with the modified Gompertz model. Therefore, the modified Gompertz model will be applicable to fit the degradation curve of various substrates by pure cultures with relatively high concentrations.
3.5 DEP-induction on microbial growth and degrada- tion rate
In this work, the effect of DEP induction on microbial biomass and degradation by Acinetobacter sp. JDC-16 was further investigated. As shown in Fig.5, the induced cells turn into logarithmic phase immediately after 6 h and the microbial biomass (OD600) increases to the maximum after 21 h. Meanwhile, DEP is rapidly degraded to undetectable level in the culture medium within 18 h. On the contrary, there is an apparent lag phase observed when cells are not induced at an initial concentration of 500 mg/L of DEP and OD600 of the non-induced bacterial biomass increases to the maximum after 30 h (Fig.5). The substrate deletion curves can be well described by the modified Gompertz model with high correlation coefficient (R2>0.99). According to the calculated parameter values, the lag phase (t0=19.852 4 h) for DEP degradation by the bacteria without induction is much longer than that of the induced cells (t0=5.485 4 h), suggesting that DEP-induction can obviously shorten the lag phase. In a previous study, it was found that DMP induction could also shorten the lag phase of DMP degradation at the initial concentration of 500 mg/L by Burkholderia cepacia DA2 [28]. It is well known that the
Fig.5 Microbial biomass and DEP degradation by DEP-induced and non-induced bacterial cells of Acinetobacter sp. JDC-16: 1—DEP degradation with induction; 2—DEP degradation without induction; 3—OD600 with induction; 4—OD600 without induction
initial step in aerobic degradation of PAEs is hydrolysis of ester bond by esterase to yield monoesters and then phthalic acid. The result in this work indicates that the esterase involved in the degradation of DEP may be inducible. DEP induction improves the esterase activity to activate the utilization of DEP. Therefore, once a bacterial population is established to breakdown the substrate, the degradation rate can be accelerated immediately.
4 Conclusions
(1) A bacterial strain capable of degrading DEP is isolated from river sludge using PAEs as the sole source of carbon and energy. It is identified as Acinetobacter sp. JDC-16 through analysis of morphology, physiochemical properties and 16S rRNA gene sequence.
(2) The substrates utilization test indicates that strain JDC-16 can utilize a variety of phthalates like DMP, DEP, DBP, DOP, DIOP and the central intermediate PA, but cannot degrade DINP and another two phthalate isomers IA and TA. The degradation experiments using DEP as the model compound show that the optimal pH and temperature for DEP degradation is 8.0 and 35 ℃, respectively.
(3) Kinetics of DEP degradation at various initial concentrations ranging from 50 to 500 mg/L can be well described with the modified Gompertz model. This shows that the lag phase and the maximum transformation rate increases with the increase of DEP concentrations, suggesting that the initial concentration may play an important role in affecting the degradation of DEP. In addition, the induction of bacteria by DEP can obviously shorten the lag phase prior to the initiation of the degradation.
References
[1] LIANG Da-wei, ZHANG Tong, HE Jian-zhong. Phthalates biodegradation in the environment [J]. Applied Microbiology Biotechnology, 2008, 80(2): 183-198.
[2] KAPANEN A, STEPHEN J R, BRUGGEMANN J, KIVIRANTA A, WHITE D C, ITAVAARA M. Diethyl phthalate in compost: Ecotoxicological effects and response of the microbial community [J]. Chemosphere, 2007, 67(11): 2201-2209.
[3] MATSUMOTO M, HIRATA-KOIZUMI M, EMA M. Potential adverse effects of phthalic acid esters on human health: A review of recent studies on reproduction [J]. Regulatory Toxicology and Pharmacology, 2008, 50(1): 37-49.
[4] WANG Jing-long, LIU Ping, QIAN Yi. Microbial degradation of di-n-butyl phthalate [J]. Chemosphere, 1995, 31(9): 4051-4056.
[5] NIAZI J H, PRASAD D T, KAREGOUDAR T B. Initial degradation of dimethylphthalate by esterases from Bacillus species [J]. FEMS Microbiology Letters, 2001, 196(2): 201-205.
[6] ZENG Feng, CUI Kun-yan, XIE Zhi-yong, WU Li-na, LIU Min, SUN Guo-quan, LIN Yu-jun, LUO Dan-ling, ZENG Zun-xiang. Phthalate esters (PAEs): Emerging organic contaminants in agricultural soils in peri-urban areas around Guangzhou, China [J]. Environmental Pollution, 2008, 156(2): 425-434.
[7] MARTTINEN S K, KETTUNEN R H, RINTALA J A. Occurrence and removal of organic pollutants in sewages and landfill leachates [J]. Science of Total Environment, 2003, 301(1/2/3): 1-12.
[8] MOHAN SV, SHAILAJA S, KRISHNA MR, REDDY KB, SARMA P N. Bioslurry phase degradation of di-ethyl phthalate (DEP) contaminated soil in periodic discontinuous mode operation: Influence of bioaugmentation and substrate partition [J]. Process Biochemistry, 2006, 41(3): 644-652.
[9] CARTWRIGHT C D, OWEN S A, THOMPSON I P, BURNS R G. Biodegradation of diethyl phthalate in soil by a novel pathway [J]. FEMS Microbiology Letters, 2000, 186(1): 27-34.
[10] CHANG B V, LIAO C S, YUAN S Y. Anaerobic degradation of diethyl phthalate, di-n-butyl phthalate, and di-(2-ethylhexyl) phthalate from river sediment in Taiwan [J]. Chemosphere, 2005, 58(11): 1601-1607.
[11] HASHIZUME K, NANYA J, TODA C, YASUI T, NAGANO H, KOJIMA N. Phthalate esters detected in various water samples and biodegradation of the phthalates by microbes isolated from river water [J]. Biological and Pharmaceutical Bulletin, 2002, 25(2): 209- 214.
[12] CHANG B V, YANG C M, CHENG C H, YUAN S Y. Biodegradation of phthalate esters by two bacteria strains [J]. Chemosphere, 2004, 55(4): 533-538.
[13] ZENG Feng, CUI Kun-yan, LI Xiang-dong, FU Jia-mo, SHENG Guo-ying. Biodegradation kinetics of phthalate esters by Pseudomonas fluoresences FS1 [J]. Process Biochemistry, 2004, 39(9): 1125-1129.
[14] HERBERT H P, LIANG D W, ZHANG T. Aerobic degradation of diethyl phthalate by Sphingomonas sp [J]. Bioresource Technology, 2007, 98(3): 717-720.
[15] CHAO W L, LIN C M, SHIUNG I I, KUO Y. Degradation of dibutyl-phthalate by soil bacteria [J]. Chemosphere, 2006, 63(8): 1377-1383.
[16] DONG Xiu-zhu, CAI Miao-ying. Manual of common determinative bacteriology [M]. Beijing: Science Press, 2001: 188-189. (in Chinese)
[17] YANG Yu, DIAO Meng-xue, SHI Wu-yang, LI Li, DAI Qin-yun, QIU Guan-zhou. Isolation and characterization of organic-sulfur degradation bacterial strain [J]. Journal of Central South University of Technology, 2007, 14(3): 324-329.
[18] ZHOU Hong-bo, LIU Xi, FU Bo, QIU Guan-zhou, HUO Qiang, ZENG Wei-min, LIU Jian-she, CHEN Xin-hua. Isolation and characterization of Acidithiobacillus caldus from several typical environments in China [J]. Journal of Central South University of Technology, 2007, 14(2): 163-169.
[19] VAMSEE-KRISHNA C, MOHAN Y, PHALE P S. Biodegradation of phthalate isomers by Pseudomonas aeruginosa PP4, Pseudomonas sp PPD and Acinetobacter lwoffii ISP4 [J]. Applied Microbiology Biotechnology, 2006, 72(6): 1263-1269.
[20] WANG Ying, TIAN Ye, HAN Bin, ZHAO Hua-bing, BI Jian-nan, CAI Bao-li. Biodegradation of phenol by free and immobilized Acinetobacter sp. strain PD12 [J]. Journal of Environmental Sciences, 2007, 19(2): 222-225. (in Chinese)
[21] ADAV S S, CHEN M Y , LEE D J, REN N Q. Degradation of phenol by Acinetobacter strain isolated from aerobic granules [J]. Chemosphere, 2007, 67(8): 1566-1572.
[22] THANGARAJ K, KAPLEY A, PUROHIT H J. Characterization of diverse Acinetobacter isolates for utilization of multiple aromatic compounds [J]. Bioresource Technology, 2008, 99(7): 2488-2494.
[23] ISHII S, KOKI J, UNNO H, HORI K. Two morphological types of cell appendages on a strongly adhesive bacterium, Acinetobacter sp. strain Tol 5 [J]. Applied Environmental Microbiology, 2004, 70(8): 5026-5029.
[24] LI Jia-xi, GU Ji-Dong, PAN Li. Transformation of dimethyl phthalate, dimethyl isophthalate and dimethyl terephthalate by Rhodococcus rubber Sa and modeling the processes using the modified Gompertz model [J]. International Biodeterioration Biodegradation, 2005, 55(3): 223-232.
[25] FAN Yan-zhen, WANG Ying-ying, QIAN Pei-yuan, GU Ji-Dong. Optimization of phthalic acid batch biodegradation and the use of
modified Richards model for modeling degradation [J]. International Biodeterioration Biodegradation, 2004, 53(1): 57-63.
[26] WANG Jing-long, ZHAO Xuan, WU Wei-zhong. Biodegradation of phthalic acid esters (PAEs) in soil bioaugmented with acclimated activated sludge [J]. Process Biochemistry, 2004, 39(12): 1837- 1841.
[27] XU Xiang-rong, LI Hua-bin, GU Ji-dong. Metabolism and biochemical pathway of n-butyl benzyl phthalate by Pseudomonas fluorescens B-1 isolated from a mangrove sediment [J]. Ecotoxicology Environmental Safety, 2007, 68(3): 379-385.
[28] WANG Ya-li, YIN Bo, HONG Yi-guo, YAN Yan, GU Ji-dong. Degradation of dimethyl carboxylic phthalate ester by Burkholderia cepacia DA2 isolated from marine sediment of South China Sea [J]. Ecotoxicology, 2008, 17(8): 845-852.
(Edited by CHEN Wei-ping)
Foundation item: Project(30770388) supported by the National Natural Science Foundation of China
Received date: 2009-11-29; Accepted date: 2010-04-26
Corresponding author: WU Xue-ling, PhD, Professor; Tel: +86-731-88804873; E-mail: xueling0714@yahoo.com.cn
- Aerobic biodegradation of diethyl phthalate by Acinetobacter sp. JDC-16 isolated from river sludge
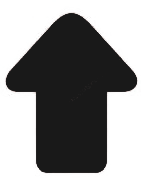