- Abstract:
- 1 Introduction▲
- 2 Experimental▲
- 3 Results and discus...▲
- 4 Conclusions▲
- References
- Figure
- Figure 1 SEM micrographs of hopeite coatings by PLD (a, b) and hopeite coatings by RF-MS (c, d)
- Figure 2 XRD patterns of hopeite target and annealed hopeite coatings deposited by PLD and RF-MS
- Figure 3 Surface topography of deposited:
- Figure 4 Schematic assembly of adhesive testing
- Figure 5 FACS graphs of pristine specimen (a), PLD hopeite coated specimen (b) and MS hopeite coated specimen (c)
- Figure 6 SEM micrographs of PLD hopeite coated specimen (a, b) and RF-MS hopeite coated specimen after immersion test (c, d)
- Figure 7 Contact angle measurement:
J. Cent. South Univ. (2020) 27: 2198-2209
DOI: https://doi.org/10.1007/s11771-020-4441-8
Multifunctional hopeite nanocoating on Ti64 substrates by pulsed laser deposition and radio frequency magnetron sputtering for orthopedic implant applications: A comparative study
Ashish DAS1, 2, Mukul SHUKLA1, 3, 4
1. Department of Mechanical Engineering, Motilal Nehru National Institute of Technology,Allahabad 211004, India;
2. Department of Production and Industrial Engineering, National Institute of Technology,Jamshedpur 831014, India;
3. School of Mechanical and Aerospace Engineering, Queen’s University, Belfast BT7 1NN,United Kingdom;
4. Department of Mechanical Engineering Technology, University of Johannesburg,Johannesburg PO Box 524, South Africa
Central South University Press and Springer-Verlag GmbH Germany, part of Springer Nature 2020
Abstract:
Functionalized implants demonstrate an upgraded approach in orthopedic implants, aiming to achieve long term success through improved bio integration. Bioceramic coatings with multifunctionality have arisen as an effective substitute for conventional coatings, owing to their combination of various properties that are essential for bio-implants, such as osteointegration and antibacterial character. In the present study, thin hopeite coatings were produced by Pulsed laser deposition (PLD) and radio frequency magnetron sputtering (RFMS) on Ti64 substrates. The obtained hopeite coatings were annealed at 500 °C in ambient air and studied in terms of surface morphology, phase composition, surface roughness, adhesion strength, antibacterial efficacy, apatite forming ability, and surface wettability by scanning electron microscope (SEM), X-ray diffraction (XRD), atomic force microscope (AFM), tensometer, fluorescence-activated cell sorting (FACS), simulated body fluid (SBF) immersion test and contact angle goniometer, respectively. Furthermore, based on promising results obtained in the present work it can be summarized that the new generation multifunctional hopeite coating synthesized by two alternative new process routes of PLD and RFMS on Ti64 substrates, provides effective alternatives to conventional coatings, largely attributed to strong osteointegration and antibacterial character of deposited hopeite coating ensuring the overall stability of metallic orthopedic implants.
Key words:
hopeite; Ti64 alloy; pulsed laser deposition; magnetron sputtering; coating; implant;
Cite this article as: Ashish DAS, Mukul SHUKLA. Multifunctional hopeite nanocoating on Ti64 substrates by pulsed
laser deposition and radio frequency magnetron sputtering for orthopedic implant applications: A comparative study [J]. Journal of Central South University, 2020, 27(8): 2198-2209. DOI: https://doi.org/10.1007/s11771-020-4441-8.
1 Introduction
Titanium alloys are largely used as bio-implants in orthopedic and dental applications owing to their non-toxic character, high specific strength and superior biocompatibility [1]. Titanium alloys, especially Ti6Al4V systems, have some serious issues of bio-inertness and release of aluminum and vanadium metal ions, which affects the bone healing process [2-6]. To overcome these issues, researchers have tried to engineer the implant’s topography and surface composition [7]. In view of shortening the bone healing period and increasing the clinical success of Ti implants, various surface modification methods have been used [8]. As reported in Ref. [9], a bioceramic coating can avert the toxic metal ions released from the implant surface into the environment. Similarly, a new generation multifunctional bioceramic coating of hopeite (hydrated zinc phosphate) can check the release of toxic ions from the surface of titanium alloys and ensure overall better bioactivity, largely because of the presence of phosphate that is the key element of osteoconductive ceramics and zinc that is a well-known element for improving biomineralization [10-12]. Hopeite (Zn3(PO4)2·4H2O), a contender bio-implant material mainly used in dental implants, can also promote bone growth [13, 14]. Hopeite formation offers low solubility, better adhesion, superior biocompatibility and excellent durability of dental cement [14-17]. As reported in Refs. [13, 18], the hopeite layer effectively promotes hydroxyapatite (HA) regrowth on the hopeite layer in the simulated body fluid (SBF) environment. SBF is a solution with an ion concentration close to that of human blood plasma. HA regrowth is credited to the high concentration of calcium and phosphorus ions, which provides a favorable condition for HA nucleation [19]. Previously conducted research has confirmed the apatite formation on bioactive material’s surface in the SBF environment, while the bulk remains unaffected [20-29]. Hopeite coating is therefore expected to improve the overall biological activity of metallic implants, including titanium alloys.
Nowadays, various surface modification techniques are reported in available literature, such as plasma spraying [30], electrochemical [31-33], electro-deposition [34, 35], pulsed laser deposition [36], electrophoretic deposition [37], ion- implantation [38], sol-gel [39, 40] and direct laser melting [41]. The pulsed laser deposition (PLD) and magnetron sputtering (MS) have arisen as an effective substitute to the above-mentioned approaches, due to their flexible characters in the deposition of thin films with desired properties [35-51]. One of the main limitations of these two methods is that they required post-deposition annealing [52, 53]. In light of the above, the goal of this study is to improve the overall biological activity of Ti64 by synthesizing a new generation multi-functional bioceramic hopeite coating using PLD and radio frequency magnetron sputtering (RFMS). To the best of our knowledge, this is a first comparative study conducted on the deposition of multifunctional hopeite coatings on Ti64 using the PLD and RF-MS techniques. This comparative study provides clear understanding, for selecting better route for fabricating a bio-implant material with improved bactericidal character, superior osseointegration, and favorable biological activity, leading to rapid healing, better stability and extended life of Ti64 implants for possible orthopedic implant applications.
2 Experimental
2.1 Pulsed laser deposition
The basic working principle and construction of the PLD method have been described elsewhere [54, 55]. Synthesis of new generation hopeite films was carried out using a PLD unit (Excel Instruments-Model: PLD-STD-12). Bioceramic hopeite targets with a diameter of 25 mm and a thickness of 3 mm were prepared by compressing the hopeite powder supplied by Asha Enterprise, Mumbai, India, with the following properties (color=white, zinc content=49.8%, phosphate= 45.8%, pH value=5.9, oil absorption (g/100 g)=32, moisture=1%, solubility=0.15%, mesh size=100% passing 100 mesh, purity≥99%) on a universal testing machine (UTM) by applying load of 10 t, followed by sintering in a furnace at 600 °C for 2 h [55]. The Ti64 alloy sheet was used as a substrate. In view of removing the superficial contaminants, the substrates were cleaned using acetone before fixing them inside the deposition chamber. In light of the literature review, the preliminary trials deposition parameters were selected as follows: deposition time=60 min, gas flow=30 cm3/min, energy fluence=2.5 J/cm2, laser energy=100 mJ, pulse frequency=10 Hz and pulse , substrate temperature=500 °C, target and substrate distance=40 mm, base pressure=10-4 Pa and deposition pressure=11 Pa [45, 46, 55-59]. Target was rotated at 15 r/min to avoid its piercing by laser striking. The as-deposited hopeite coatings have a thickness value of (249±4) nm, measured by an ellipsometer (J. A. Woollam Co. Inc.-Model: V-VASE). Obtained coatings were thermally treated in a furnace at 500 °C in ambient air for 2 h to preserve the initial crystalline status of produced coatings.
2.2 Magnetron sputtering
The basic working principle and construction of the MS method have been described elsewhere [60-63]. Synthesis of new generation hopeite films was done using a RFMS unit (Hind High Vacuum Co. (P) Ltd, Model: RF/DC sputtering unit). Bioceramic hopeite targets (50 mm diameter and 2 mm thickness) were prepared by compressing the hopeite powder (supplied by Asha Enterprise, Mumbai, India, with the following properties (color=white, zinc content=49.8%, phosphate= 45.8%, pH value=5.9, oil absorption (g/100 g)=32, moisture=1%, solubility=0.15%, mesh size=100% passing 100 mesh, purity≥99%) on a UTM by applying load of 10 t, followed by sintering in a furnace at 600 °C for 2 h [55]. The Ti64 alloy sheet was used as a substrate. In view of removing the superficial contaminants, the substrates were cleaned using acetone before fixing them (in a group of four) inside the deposition chamber. In light of the literature review and preliminary trials, the deposition parameters were selected as follows: deposition time=90 min, chamber pressure= 3.5 Pa, target fed power=200 W, substrate temperature= room temperature, argon flow rate= 15 cm3/min, base pressure=5.8×10-4 Pa, target and substrate distance= 5 cm [60, 61, 63-65]. The as-deposited hopeite coatings have a thickness value of (216±5) nm, measured by an ellipsometer (J. A. Woollam Co. Inc.-Model: V-VASE). Obtained coatings were thermally treated in a furnace at 500 °C in ambient air for 2 h to preserve the initial crystalline status of produced coatings.
Thereafter the PLD and RF-MS hopeite films were studied in terms of superficial morphology, phase composition, surface roughness, adhesion strength, antibacterial efficacy, apatite forming ability, and surface wettability by scanning electron microscopy (SEM) (ZEISS Model: EVO15), X-ray diffraction (XRD) (RIGAKU Model: Smart lab 3 kW); the operating conditions were 40 kV and 30 mA with Cu Kα radiation, atomic force microscopy (AFM) (Agilent Technologies Model: 5500), Tinius Olsen tensometer, FACS technique, one of the best cell sorting technology [66], SBF immersion and contact angle goniometer.
3 Results and discussion
3.1 SEM analysis of hopeite coatings
Figure 1 reveals the surface morphology of as-deposited PLD and RF-MS hopeite coatings.
Figure 1 SEM micrographs of hopeite coatings by PLD (a, b) and hopeite coatings by RF-MS (c, d)
PLD hopeite coatings exhibit irregular morphology consisting of spherical shape with particulates size range of 0.5-5 μm in diameter. PLD hopeite coatings are crack-free, being free of other defects and covering the entire substrate surface. Specific areas of the substrate have a greater number of deposited particles, which can be credited to the fluence used [67], which leads to an increase in the roughness of coating. By contrast, RF-MS hopeite coating was smooth (representative of the magnetron sputtering deposited structure) [68]. Furthermore, the coating is dense in character, crack-free, free of other defects and covers the entire substrate surface. Grinding of the substrate results in parallel grooved lines, which are clearly evident on the surface. Similar superficial morphology was reported by THIAN et al [69]. Specific areas of the substrate have a large number of deposited particles, which can be attributed to high ionic energies [62], which leads to an increase in the roughness of coating. Higher surface roughness obtained is due to a large number of deposited particles, leading to superior ossification [70-72], largely essential for biomedical applications.
3.2 XRD analysis of hopeite coatings
Crystalline XRD peaks of hopeite target and thermally treated hopeite coatings are clearly visible in Figure 2. Furthermore, a significant increase in the crystallinity of PLD and RF-MS hopeite coating was about 90% and 75%, respectively in intensity at 2θ=40.44° of hopeite peak after post-deposition annealing, which was also confirmed from the literature too [55]. The obtained results of X-ray diffractograms are in line with Ref. [73], ensuring the existence of the crystalline hopeite phase in the coating along with some minor traces of the amorphous phase. Throughout the deposition, no stoichiometric changes were observed. Bioceramic coating crystallinity is directly linked with its solubility (i.e. durability) of coatings in the physiological environment. Hence for a mineral coating to perform optimally in vivo, it must be crystalline in nature [63].
Figure 2 XRD patterns of hopeite target and annealed hopeite coatings deposited by PLD and RF-MS
3.3 AFM analysis of hopeite coatings
Figure 3 shows surface topography of the deposited PLD and RF-MS hopeite coating. From the 3D morphology of the coatings, it is clearly visible that the coating surface has valley formation. These valleys are responsible for the increase in average roughness value of PLD and RF-MS coating surface to 7.28 and 3.42 nm, respectively, which is greater than the average roughness of 1.7 nm reported by LOPEZ et al [74]. It is already proven that human osteoblasts tend to adhere more easily to surfaces having roughness less than 500 nm in comparison to surfaces with a roughness greater than 2000 nm [75]. Furthermore, bone tissues have inherent nanoscale topographies and roughness due to their hierarchical micron-to-nanostructures. Biologically inspired nanostructured materials have been shown to enhance tissue and bone cell responses and promote the efficacy of implants. Nanoscale surface roughness has increased osteoblast adhesion, cell proliferation compared to the same chemistry of larger scales [76-78]. The increased adsorption of cell adhesive proteins on nanoscale rough surfaces is attributed to uneven surface landscapes and increased specific surface area (resulting in significantly increase in material surface reactivity or surface affinity for the adsorption of proteins or biological entities, leading to enhanced cell adhesion) [79, 80]. Hence, human osteoblasts are likely to adhere easily to the surface of hopeite coatings fabricated in this research work.
Figure 3 Surface topography of deposited:
3.4 Adhesive strength of hopeite coatings
Tensile pull out experiments is conducted (according to ASTM C633) on a Tinius Olsen tensiometer to find out the bonding strength of deposited hopeite coatings. A schematic assembly of adhesive testing is shown in Figure 4, which includes a Ti64 stud attached to the hopeite coated surface using epoxy adhesive, cured for 48 h at room temperature. After the installation of assembly on the tensometer, pulling of the stud is carried out in tensile mode until failure. Tensile pullout experiments reveal that the adhesive strength values of PLD and MS hopeite coatings are (17.25±1.7) MPa and (21.15±2.5) MPa, respectively [62],which were better than the published value of (13.05±2.2) MPa [71, 72].
Figure 4 Schematic assembly of adhesive testing
3.5 Antibacterial efficacy of hopeite coatings
In order to confirm the reattainment of antibacterial property in the hopeite coatings deposited by PLD and RFMS technique, the FACS study was conducted to investigate the in vitro antibacterial activity of the pristine and coated specimens, against Escherichia coli (E. coli). This is necessary to overcome the skin-seal problem in implants [81]. Specimen preparation and procedure for conducting the test implemented in this examination were followed precisely, as specified in Refs. [58, 65, 82-84].
FACS results are shown in Figure 5 in terms of forward scattering (FSC) and side scattering (SSC) values, which indicates a decrease in size (confirmed by FSC values) and granularity (confirmed by SSC values) for PLD hopeite coated specimen (FSC=41710.07; SSC=13118.48) and RF-MS hopeite coated specimen (FSC=42471.25; SSC=12773.34) in comparison to pristine specimen (FSC=50162.66; SSC=15529.52). The attained results confirm death of E. coli’s in the presence of hopeite coated specimen. This bactericidal character of coating is essential for reducing bacterial adhesion and enhancing ossification [85].
3.6 Immersion test of hopeite coatings
SBF immersion experiments were performed to study the biological activity of deposited hopeite coatings. SBF immersion test was performed for 28 d following the previously reported procedure [86, 87]. Post SBF immersion SEM analysis (Figure 6) confirms apatite layer growth on the surface of hopeite coating, responsible for a very slight increase in weight of PLD hopeite coated substrate and RF-MS coated substrate, nearly 0.15% and 0.13%, respectively, after immersion test [88]. Previously conducted studies have confirmed that apatite layer growth on the surface of bioactive materials is essential for establishing superior implant-tissue interface interaction [89]. The process of apatite growth is attributed to the presence of negative species (PO43-) on the surface of hopeite coating [90], which promotes the adsorption of Ca2+ from SBF solution [13], resulting in the formation of tri-calcium phosphate (TCP) as stated by the following reaction:
Figure 5 FACS graphs of pristine specimen (a), PLD hopeite coated specimen (b) and MS hopeite coated specimen (c)
Zn3(PO4)2+3Ca2+→Ca3(PO4)2+3Zn2+
Afterward, TCP reacts with water molecules to form HA, as demonstrated by the following standard reaction:
4Ca3(PO4)2+H2O→Ca10(PO4)6(OH)2+2Ca2++2HPO42-
which is already well established by GU et al [91]. The rapid apatite growth on the superficies of hopeite coating indicates that the coating fabricated in this research work is more bioactive than the pristine Ti64 implant and hence superior osseointegration can be achieved [85].
3.7 Surface wettability of hopeite coatings
In order to evaluate the surface wettability, test was conducted according to ASTM D7334-08 using (contact angle goniometer). In surface specimens, a drop of water of (1±0.1) μL was used to measure the contact angles in a specific time (5 s).
The obtained results of the test were represented in Figure 7, which clearly shows that the PLD and RF-MS hopeite coated specimens are hydrophobic in character (average contact angle= 133.9° and 130.95°, respectively) in comparison to hydrophilic character of uncoated specimens (average contact angle=88.7°). Surfaces with hydrophilic character exhibit low interfacial free energy, which reduces ossification [92-94]. Therefore, the hopeite coating fabricated in this research enhances ossification, ensured by the formation of an apatite layer on the hopeite coated surface as shown in Figure 7.
4 Conclusions
The present research work provides clear understanding, for selecting the better deposition technique for fabricating a bio-implant material with improved bactericidal character, superior osseointegration and favorable biological activity, leading to rapid healing, better stability and extended life of Ti64 implants for possible orthopedic implant applications. SEM micrograph reveals that specific areas of the substrate have no more of deposited particles. Excess particles on the surface are desirable for biomedical applications because they promote cell growth and proliferation. X-ray diffractograms confirmed the existence of the crystalline hopeite phase in the coating which is essential for the optimal in vivo performance of hopeite coating. FACS results confirm the death of E. coli’s in the presence of hopeite coated specimen. The rapid apatite growth on the surface of hopeite coating indicates the coating fabricated in this research work is more bioactive than the pristine Ti64 implant and hence superior osseointegration can be achieved.
Figure 6 SEM micrographs of PLD hopeite coated specimen (a, b) and RF-MS hopeite coated specimen after immersion test (c, d)
Figure 7 Contact angle measurement:
Acknowledgements
We express our sincere thanks to MNNIT Allahabad, IIT Kanpur, RRCAT Indore, MHRD GOI and UOJ South Africa for their valuable support during the entire duration of the study.
References
[1] YANG W H, XI X F, SI Y, HUANG S, WANG J F, CAI K Y. Surface engineering of titanium alloy substrates with multilayered biomimetic hierarchical films to regulate the growth behaviors of osteoblasts [J]. Acta Biomaterialia, 2014, 10(10): 4525-4536. DOI: 10.1016/j.actbio.2014.05.033.
[2] HALLAB N J, VERMES C, MESSINA C, ROEBUCK K A, GLANT T T, JACOBS J J. Concentration and composition dependent effects of metal ions on human MG-63 osteoblasts [J]. Journal of Biomedical Materials Research, 2002, 60(3): 420-433. DOI: 10.1002/jbm.10106.
[3] SUN Z L, WATAHA J C, HANKS C T. Effects of metal ions on osteoblast like cell metabolism and differentiation [J]. Journal of Biomedical Materials Research, 1997, 34(1): 29-37. DOI: 10.1002/(sici)1097-4636(199701)34:1<29::aid- jbm5>3.0.co;2-p.
[4] THOMPSON G J, PUELO D A. TC4 ion solution inhibition of osteogenic cell phenotype as a function of differentiation time course in vitro [J]. Biomaterials, 1996, 17(20): 1949-1954. DOI: 10.1016/0142-9612(96)00009-9.
[5] WANG J Y, WICKLUND B H, GUSTILO R B, TSUKAYAMA D T. Prosthetic metals interfere with the functions of human osteoblast cells in vitro [J]. Clinical Orthopaedics and Related Research, 1997, 339: 216-226. DOI: 10.1097/00003086-199706000-00030.
[6] MOHAMMED M T, KHAN Z A, SIDDIQUEE A N. Titanium and its alloys the imperative materials for biomedical applications [C]// ICRTET. Meerut, India, 2012: 91-95.
[7] YU J, CHU X, CAI Y, TONG P, YAO J. Preparation and characterization of antimicrobial nano hydroxyapatite composites [J]. Materials Science and Engineering C, 2014, 37: 54-59. DOI: 10.1016/j.msec.2013.12.038.
[8] PARK J W, PARK K B, SUH J Y. Effects of calcium ion incorporation on bone healing of Ti6Al4V alloy implants in rabbit tibiae [J]. Biomaterials, 2007, 28(22): 3306-3313. DOI: 10.1016/j.biomaterials.2007.04.007.
[9] SRIDHAR T M, ARUMUGAM T K, RAJESWARI S, SUBBAIYAN M. Electrochemical behaviour of hydroxyapatite-coated stainless-steel implants [J]. Journal of Materials Science Letters, 1997, 16(22): 1964-1966. DOI: 10.1023/A:1018511406374.
[10] YAMAGUCHI M, OISHI H, SUKETA Y. Stimulatory effect of zinc on bone formation in tissue culture [J]. Journal of Materials Science Letters, 1987, 36(22): 4007-4012. DOI: 10.1016/0006-2952(87)90471-0.
[11] EBERLE J, SCHMIDMAYER S, ERBEN R G, STANGASSINGER M, ROTH H P. Skeletal effects of zinc deficiency in growing rats [J]. Journal of Trace Elements in Medicine and Biology, 1999, 13(1, 2): 21-26. DOI: 10.1016/ S0946-672X (99)80019-4.
[12] CHEN D, WAITE L C, PIERCE W M. In vitro effects of zinc on markers of bone formation [J]. Biological Trace Element Research, 1999, 68(3): 225-234. DOI: 10.1007/BF02783905.
[13] SHIBLI S M A, JAYALEKSHMI A C. Development of phosphate inter layered hydroxyapatite coating for stainless steel implant [J]. Applied Surface Science, 2008, 254(13): 4103-4110. DOI: 10.1016/j.apsusc.2007.12.05.
[14] HERSCHKE L, ROTTSTEGGE J, LIEBERWIRTH I, WEGNER G. Zinc phosphate as versatile material for potential biomedical applications, Part 1 [J]. Journal of Materials Science: Materials in Medicine, 2006, 17(1): 81-94. DOI: 10.1007/s10856-006-6332-4.
[15] NRIAGU J O. Solubility equilibrium constant of α-hopeite [J]. Geochimica et Cosmochimica Acta, 1973, 37(6): 2357-2361. DOI: 10.1016/0016-7037(73)90 284-6.
[16] UO M, SJOREN G, SUNDH A, WATARI F, BERGMAN M, LERNER U. Cytotoxicity and bonding property of dental ceramics [J]. Dental Materials, 2003, 19(6): 487-492. DOI: 10.1016/s0109-5641(02)00094-5.
[17] ATTAR N, TAM L E, MCCOMB D. Mechanical and physical properties of contemporary dental luting agents [J]. Journal of Prosthetic Dentistry, 2003, 89(2): 127-134. DOI: 10.1067/mpr.2003.20.
[18] HORIUCHI S, ASAOKA K, TANAKA E. Development of a novel cement by conversion of hopeite in set zinc phosphate cement into biocompatible apatite [J]. Bio-Medical Materials and Engineering, 2009, 19(2, 3): 121-131. DOI: 10.3233/ BME-2009-0571.
[19] LIN F H, HSU Y S, LIN S H, SUN J S. The effect of Ca/P concentration and temperature of simulated body fluid on the growth of hydroxyapatite coating on alkali-treated 316L stainless steel [J]. Biomaterials, 2002, 23(19): 4029-4038. DOI: 10.1016/S0142-9612(02)00154-0.
[20] KOKUBO T, ITO S, SAKKA S, YAMAMURO T. Formation of a high strength bioactive glass-ceramic in the system MgO-CaO-SiO2-P2O5 [J]. Journal of Materials Science, 1986, 21: 536-540. DOI: 10.1007/BF01145520.
[21] KOKUBO T, HAYASHI T, SAKKA S, KITSUGI T, YAMAMURO T. Bonding between bioactive glasses, glass-ceramics or ceramics in simulated body fluid [J]. Journal of the Ceramic Association, 1987, 95: 785-791. DOI: 10.2109/jcersj1950.95.1104_ 785.
[22] KOKUBO T, KUSHITANI H, SAKKA S, KITSUGI T, YAMAMURO T. Solutions able to reproduce in vivo surface-structure changes in bioactive glass–ceramics A-W3 [J]. Journal of Biomedical Materials Research, 1990, 24(6): 721-734. DOI: 10.1002/jbm.820240607.
[23] KOKUBO T. Surface chemistry of bioactive glass–ceramics [J]. Journal of Non-Crystalline Solids, 1990, 120(1-3): 138-151. DOI: 10.1016/0022-3093(90)90199-V.
[24] AZA P N D, GUITIAN F, AZA S D. Bioactivity of wollastonite ceramics: In vitro evaluation [J]. Scripta Metallurgica et Materialia, 1994, 31: 1001-1005. DOI: 10.1016/0956-716X(94)90517-7.
[25] LIU D M. Bioactive glass-ceramic: Formation, characterization and bioactivity [J]. Materials Chemistry and Physics, 1994, 36: 294-303. DOI: 10.1016/0254-0584(94) 90045-0.
[26] AZA P N D, LUKLINSKA Z B, ANSEAU M R, GUITIAN F, AZA S D E. Bioactivity of pseudowollastonite in human saliva [J]. Journal of Dentistry, 1999, 27: 107-113. DOI: 10.1016/S0300-5712(98)00029-3.
[27] AZA P N D, LUKLINSKA Z. Effect of the glass-ceramic microstructure on its in vitro bioactivity [J]. Journal of Materials Science: Materials in Medicine, 2003, 14: 891-898. DOI: 10.1023/A:1025686727291.
[28] AZA P N D, LUKLINSKA Z B, ANSEAU M R. Bioactivity of diopside ceramic in human parotid saliva [J]. Journal of Biomedical Materials Research, 2005, 73: 54-60. DOI: 10.1002/jbm.b.30187.
[29] ALEMANY M I, VELASQUEZ P, de la CASA-LILLO M A, AZA P N D. Effect of materials processing methods on the in vitro bioactivity of wollastonite glass-ceramic materials [J]. Journal of Non-Crystalline Solids, 2005, 351: 1716-1726. DOI: 10.1016/j.jnoncrysol.2005. 04.062.
[30] KHAN A N, LU J. Thermal cyclic behavior of air plasma sprayed thermal barrier coatings sprayed on stainless steel substrates [J]. Surface and Coatings Technology, 2007, 201(8): 4653-4658. DOI: 10.1016/j.surfcoat.2006.10.022.
[31] OSKUIE A A, AFSHAR A, HASANNEJAD H. Effect of current density on DC electrochemical phosphating of stainless steel 316 [J]. Surface and Coatings Technology, 2010, 205(7): 2302-2306. DOI: 10.1016/j.surfcoat.2010.09. 016.
[32] IONITA D, UNGUREANU C, DEMETRESCU I. Electrochemical and antibacterial performance of CoCrMo alloy coated with hydroxyapatite or silver nanoparticles [J]. Journal of Materials Engineering and Performance, 2013, 22: 3584-3591. DOI: 10.1007/s11665-013-0653-5.
[33] KAVITHA C, NARAYANAN T S N S, RAVICHANDRAN K, PARK I L S, LEE M H. Deposition of zinc-zinc phosphate composite coatings on steel by cathodic electrochemical treatment [J]. Journal of Coatings Technology and Research, 2014, 11(3): 431-442. DOI: 10.1007/s11998-013-9533-z.
[34] VALANEZHAD A, TSURU K, MARUTA M, KAWACHI G, MATSUYA S, ISHIKAWA K. Zinc phosphate coating on 316L-type stainless steel using hydrothermal treatment [J]. Surface and Coatings Technology, 2010, 205(7): 2538-2541. DOI: 10.1016/j.surfcoat.2010.09.050.
[35] RAD H R B, HAMZAH E, KADIR M R A, SAUD S. The mechanical properties and corrosion behavior of double-layered nano hydroxyapatite-polymer coating on Mg-Ca alloy [J]. Journal of Materials Engineering and Performance, 2015, 24: 4010-4021. DOI: 10.1007/s11665- 015-1661-4.
[36] CARRADO A. Nano-crystalline pulsed laser deposition hydroxyapatite thin films on Ti substrate for biomedical application [J]. Journal of Coatings Technology and Research, 2011, 8: 749-755. DOI: 10.1007/s11998-011- 9355-9.
[37] KHALILI V, ALLAFI J K, GHALEH H M, PAULSEN A, FRENZEL J, EGGELER G. The influence of Si as reactive bonding agent in the electrophoretic coatings of HA-Si-MWCNTs on NiTi alloys [J]. Journal of Materials Engineering and Performance, 2016, 25(2): 390-400. DOI: 10.1007/s11665-015-1824-3.
[38] FOERSTER C E, SERBENA F C, DA SILVA S L R, LEPIENSKI C M, DE M, SIQUEIRA C J, UEDA M. Mechanical and tribological properties of AISI 304 stainless steel nitrided by glow discharge compared to ion implantation and plasma immersion ion implantation [J]. Nuclear Physics B, 2007, 257(1, 2): 732-736. DOI: 10.1016/ j.nimb.2007.01.266.
[39] KHEIMEHSARI H, IZMAN S, SHIRDAR M R. Effects of HA-coating on the surface morphology and corrosion behavior of a Co-Cr-based implant in different conditions [J]. Journal of Materials Engineering and Performance, 2015, 24(6): 2294-2302. DOI: https://link.springer.com/article/ 10.1007/s11665-015-1517-y.
[40] AKSAKAL B, GAVGALI M, DIKICI B. The effect of coating thickness on corrosion resistance of hydroxyapatite coated Ti6Al4V and 316L SS implants [J]. Journal of Materials Engineering and Performance, 2010, 19(6): 894-899. DOI: https://link.springer.com/article/10.1007/ s11665-009-9559-7.
[41] TLOTLENG M, AKINLABI E, SHUKLA M, PITYANA S. Microstructures, hardness and bioactivity of hydroxyapatite coatings deposited by direct laser melting process [J]. Materials Science and Engineering C, 2014, 43: 189-198. DOI: 10.1016/j.msec.2014.06.032.
[42] GARCIA S F J, MAYOR M B, ARIAS J L, POU J, LEON B, PEREZ A M. Hydroxyapatite coatings: A comparative study between plasmaspray and pulsed laser deposition techniques [J]. Journal of Materials Science: Materials in Medicine, 1997, 8(12): 861-865. DOI: 10.1023/A:1018549720873.
[43] BAO Q, CHEN C, WANG D, JI Q, LEI T. Pulsed laser deposition and its current research status in preparing hydroxyapatite thin films [J]. Applied Surface Science, 2005, 252(5): 1538-1544. DOI: 10.1016/j.apsusc. 2005.02.127.
[44] BIGI A, BRACCI B, CUISINIER F, ELKAIM R, FINI M, MAYER I, MIHAILESCU IN, SOCOL G, STURBA L, TORRICELLI P. Human osteoblast response to pulsed laser deposited calcium phosphate coatings [J]. Biomaterials, 2005, 26(15): 2381-2389. DOI: 10.1016/j.biomaterials.2004.07. 057.
[45] FERNANDEZ P J M, GARCIA C M V, CLERIES L, SARDIN G, MORENZA J L. Influence of the interface layer on the adhesion of pulsed laser deposited hydroxyapatite coatings on titanium alloy [J]. Applied Surface Science, 2002, 195(1-4): 31-37. DOI: 10.1016/S0169-4332(02)00002-8.
[46] ZENG H, LACEFIELD W R. XPS, EDX and FTIR analysis of pulsed laser deposited calcium phosphate bioceramic coatings: The effects of various process parameters [J]. Biomaterials, 2000, 21(1): 23-30. DOI: 10.1016/S0142- 9612(99)00128-3.
[47] RAJESH P, MURALEEDHARAN C V, KOMATH M, VARMA H. Pulsed laser deposition of hydroxyapatite on titanium substrate with titania interlayer [J]. Journal of Materials Science: Materials in Medicine, 2011, 22(3): 497-505. DOI: 10.1007/s10856-011-4230-x.
[48] WON Y J, KI H. Fabricating functionally graded films with designed gradient profiles using pulsed laser deposition [J]. Journal of Applied Physics, 2013, 113: 174910. DOI: 10.1063/1.4803692.
[49] TANASKOVIC D, JOKIC B, SOCOL G, POPESCU A, MIHAILESCU I N, PETROVIC R, JANACKOVIC D. Synthesis of functionally graded bioactive glass-apatite multistructures on Ti substrates by pulsed laser deposition [J]. Applied Surface Science, 2007, 254(4): 1279-1282. DOI: 10.1016/j.apsusc.2007.08.009.
[50] SHI J Z, CHEN C Z, YU H J, ZHANG S J. Application of magnetron sputtering for producing bioactive ceramic coatings on implant materials [J]. Bulletin of Materials Science, 2008, 31: 877-884. DOI: 10.1007/s12034-008- 0140-z.
[51] SURMENEV R A. A review of plasma-assisted methods for calcium phosphate-based coatings fabrication [J]. Surface and Coatings Technology, 2012, 206(8, 9): 2035-2056. DOI: 10.1016/j.surfcoat.2011.11.002.
[52] RUSOP M, UMA K, SOGA T, JIMBO T. Structural properties of pulsed laser deposited zinc oxide thin films annealed at various temperatures [J]. Surface Engineering, 2007, 23(3): 230-233. DOI: 10.1179/174329406X122964.
[53] YANG Y, KIM K H, ONG J L. A review on calcium phosphate coatings produced using a sputtering process-an alternative to plasma spraying [J]. Biomaterials, 2005, 26(3): 327-337. DOI: 10.1016/j.biomaterials.2004. 02.029.
[54] KUPPUSAMI P, RAGHUNATHAN V S. Status of pulsed laser deposition: Challenges and opportunities [J]. Surface Engineering, 2006, 22(2): 81-83. DOI: 10.1179/1743294 06X98502.
[55] KHANDELWAL H, SINGH G, AGRAWAL K, PRAKASH S, AGARWAL R D. Characterization of hydroxyapatite coating by pulse laser deposition technique on stainless steel 316L by varying laser energy [J]. Applied Surface Science, 2013, 265: 30-35. DOI: 10.1016/j.apsusc.2012. 10.072.
[56] ZENG H, LACEFIELD W R, MIROV S. Structural and morphological study of pulsed laser deposited calcium phosphate bioceramic coatings: Influence of deposition conditions, laser parameters, and target properties [J]. Journal of Biomedical Materials Research, 2000, 50(2): 248-258. DOI: 10.1002/(SICI)1097-4636(200005)50:2< 248::AID-JBM20>3.0.CO;2-I.
[57] DAS A, SHUKLA M. Surface morphology and adhesion studies of pulsed laser deposited hydroxyapatite thin film coatings on SS254 stainless steel [C]// 24th DAE BRNS National Laser Symposium (NLS-24), 2015.
[58] DAS A, SHUKLA M. Surface morphology, bioactivity, and antibacterial studies of pulsed laser deposited hydroxyapatite coatings on stainless steel 254 for orthopedic implant applications [J]. Journal of Materials: Design and Applications, 2016, 233(2): 120-127. DOI: 10.1177/1464 420716663029.
[59] CONSTANTINO M E, CAMPILLO B, STAIA M H, SERNA S, JUAREZ-ISLAS J, SUDARSHAN T S. Pulsed electrode deposition of super hard coatings on steel substrates: microstructural and chemical study [J]. Surface Engineering, 2006, 22(3): 212-218. DOI: 10.1179/17432 9406X108843.
[60] ZHANG S. Hydroxyapatite coatings for biomedical applications [M]. CRC Press, 2018.
[61] WASA K. Handbook of sputtering technology [M]. Elsevier, 2012. DOI: 10.1016/C2010-0-67037-4.
[62] SWANN S. Magnetron sputtering [J]. Physics in Technology, 1988, 19(2): 67-75. DOI: 10.1088/0305-4624/ 19/2/304.
[63] DRIVER M. Coatings for biomedical applications [M]. Woodhead Publishing Limited, 2012.
[64] WAN T, AOKI H, HIKAWA J, LEE J H. RF-magnetron sputtering technique for producing hydroxyapatite coating film on various substrates [J]. Bio-Medical Materials and Engineering, 2007, 17(5): 291-297. DOI: https://europepmc. org/article/med/17851171.
[65] DAS A, SHUKLA M. Surface morphology and in vitro bioactivity of biocompatible hydroxyapatite coatings on medical grade S31254 steel by RF magnetron sputtering deposition [J]. International Journal of Surface Engineering and Coatings, 2017, 95(5): 276-281. DOI: 10.1080/ 00202967.2017.1323675.
[66] WOLKOWICZ R. Fluorescence-activated cell sorting, Brenner’s encyclopedia of genetics [M]. Academic Press, 2013.
[67] KASSING R, PETKOV P, KULISCH W, POPOV C. Functional properties of nanostructured materials series II: Mathematics physics and chemistry [M]. Springer, 2006.
[68] NELEA V, MOROSANU C, ILIESCU M, MIHAILESCU I N. Hydroxyapatite thin films grown by pulsed laser deposition and radio-frequency magnetron sputtering: comparative study [J]. Applied Surface Science, 228(1): 346-356. DOI: 10.1016/j.apsusc.2004.01.029.
[69] THIAN E S, HUANG J, BEST S M, BARBER Z H, BONFIELD W. Magnetron co-sputtered silicon-containing hydroxyapatite thin films—An in vitro study [J]. Biomaterials, 2005, 26(16): 2947-2956. DOI: 10.1016/j.biomaterials.2004. 07.058.
[70] DINDA G P, SHIN J, MAZUMDER J. Pulse laser deposition of hydroxyapatite thin coatings on TC4: Effect of heat treatment on structure and properties [J]. Acta Biomaterialia, 2009, 5(5): 1821-1830. DOI: 10.1016/j.actbio.2009.01.027.
[71] BLIND O, KLEIN L H, DAILEY B, JORDAN L. Characterization of hydroxyapatite films obtained by pulsed-laser deposition on Ti and TC4 substrates [J]. Dental Materials, 2005, 21(11): 1017-1024. DOI: 10.1016/ j.dental.2004.12.003.
[72] TORRISI L. Structural investigations on laser deposited hydroxyapatite films [J]. Thin Solid Films, 1994, 237(1, 2): 12-15. DOI: 10.1016/0040-6090(94)90229-1.
[73] ZHANG X, XIAO G Y, JIAO Y, ZHAO X C, LU Y P. Facile preparation of hopeite coating on stainless steel by chemical conversion method [J]. Surface and Coatings Technology, 2014, 240: 361-364. DOI: 10.1016/ j.surfcoat.2013.12.054.
[74] LOPEZ E O, MELLO A, SENDAO H, COSTA L T, ROSSI A L, OSPINA O R, BORGHI F F, FILHO J G S, ROSSI A M M. Growth of crystalline hydroxyapatite thin films at room temperature by tuning the energy of the RF-Magnetron sputtering plasma [J]. ACS Applied Materials & Interfaces, 2013, 5(19): 9435-9445. DOI: 10.1021/am4020007.
[75] ANSELME K, BIGERELLE M, NOEL B, DUFRESNE E, JUDAS D, IOST A, HARDOUIN P. Qualitative and quantitative study of human osteoblast adhesion on materials with various surface roughnesses [J]. Journal of Biomedical Materials Research, 2000, 49(2): 155-166. DOI: 10.1002/(SICI)1097-4636(200002)49:2<155::AID-JBM2>3.0.CO;2-J
[76] PRICE R L, WAID M C, HABERSTROH K M, WEBSTER T J. Selective bone cell adhesion on formulations containing carbon nanofibers [J]. Biomaterials, 2003, 24(11): 1877-1887. DOI: 10.1016/S0142-9612(02)00 609-9.
[77] WASHBURN N R, YAMADA K M, SIMON C G, KENNEDY S B, AMIS E J. High-throughput investigation of osteoblast response to polymer crystallinity: Influence of nanometer-scale roughness on proliferation [J]. Biomaterials, 2004, 25(7, 8): 1215-1224. DOI: 10.1016/j.biomaterials. 2003.08.043.
[78] WEBSTER T J, HELLENMEYER E L, PRICE R L. Increased osteoblast functions on theta plus delta nanofiber alumina [J]. Biomaterials, 2005, 26(9): 953-960. DOI: 10.1016/j.biomaterials.0204.03.040.
[79] WILSON C J, CLEGG R E, LEAVESLEY D I, PEARCY M J. Mediation of biomaterial-cell interactions by adsorbed proteins: A review [J]. Tissue Engineering, 2005, 11(1, 2): 1-18. DOI: 10.1089/ten.2005.11.1.
[80] YANG L. Nanotechnology-enhanced orthopedic materials fabrications, applications and future trends [M]. Woodhead Publishing, 2015. DOI: 10.1016/C2013-0-16378-7.
[81] GE X, LENG Y, BAO C, XU S L, WANG R, REN F. Antibacterial coatings of fluoridated hydroxyapatite for percutaneous implants [J]. Journal of Biomedical Materials Research Part A, 2010, 95(2): 588-599. DOI: 10.1002/jbm.a.32862.
[82] SARITHA K, RAJESH A, MANJULATHA K, SETTY O H, YENUGU S. Mechanism of antibacterial action of the alcoholic extracts of Hemidesmus indicus (L.) R. Br. exSchult, Leucas aspera (Wild.), Plumbago zeylanica L., and Tridax procumbens (L.) R. Br. ex Schult [J]. Frontiers in Microbiology, 2015, 6: 1-9. DOI: 10.3389/fmicb.2015. 00577.
[83] DAS A, SHUKLA M. Hydroxyapatite coatings on high nitrogen stainless steel by laser rapid manufacturing [J]. Journal of the Minerals, Metals & Materials Society, 2017, 69(11): 2292-2296. DOI: 10.1007/s11837-017-2529-x.
[84] DAS A, SHUKLA M. Pulsed laser-deposited hopeite coatings on titanium alloy for orthopaedic implant applications: surface characterization, antibacterial and bioactivity studies [J]. Journal of the Brazilian Society of Mechanical Sciences and Engineering, 2019, 41(5): 214. DOI: 10.1007/s40430-019-1722-y.
[85] PUCKETT S D, TAYLOR E, RAIMONDO T, WEBSTER T J. The relationship between the nanostructure of titanium surfaces and bacterial attachment [J]. Biomaterials, 2010, 31(4): 706-713. DOI: 10.1016/j.biomaterials. 2009.09.081.
[86] YI W, SUN X, NIU D, HU X. In vitro bioactivity of 3D Ti-mesh with bioceramic coatings in simulated body fluid [J]. Journal of Asian Ceramic Societies, 2014, 2(3): 210-214. DOI: 10.1016/j.jascer.2014.04.002.
[87] KOKUBO T, TAKADAMA H. How useful is SBF in predicting in vivo bone bioactivity? [J]. Biomaterials, 2006, 27(15): 2907-2915. DOI: 10.1016/j.biomateri als.2006.01.017.
[88] MAN H C, CHIU K Y, CHENG F T, WONG K H. Adhesion study of pulsed laser deposited hydroxyapatite coating on laser surface nitrided titanium [J]. Thin Solid Films, 2009, 517(18): 5496-5501. DOI: 10.1016/j.tsf. 2009.03.208.
[89] NEO M, KOTANI S, NAKAMURA T, YAMAMURO T, OHTSUKI C, KOKUBO T, BANDO Y A. A comparative study of ultrastructures of the interfaces between four kinds of surface-active ceramic and bone [J]. Journal of Biomedical Materials Research, 1992, 26(11): 1419-1432. DOI: 10.1002/jbm.820261103.
[90] CHAVAN P N, BAHIR M M, MENE R U, MAHABOLE M P, KHAIRNAR R S. Study of nano biomaterial hydroxyapatite in simulated body fluid: formation and growth of apatite [J]. Material Science & Engineering B, 2010, 168(1): 224-230. DOI: 10.1016/j.mseb. 2009.11.012.
[91] GU Y W, KHOR K A, CHEANG P. Bone-like apatite layer formation on hydroxyapatiteprepared by spark plasma sintering (SPS) [J]. Biomaterials, 2004, 25(18): 4127-4134. DOI: 10.1016/j.biomaterials.2003.11.030.
[92] WANG Y X, ROBERTSON J L, SPILLMAN W B, CLAUS R O. Effects of the chemical structure and the surface properties of polymeric biomaterials and their biocompatibility [J]. Journal of Pharmaceutical Research, 2004, 21(18): 1362-1373. DOI: 10.1023/B:PHAM.00000 36909.41843.18.
[93] WANG J, PAN C J, HUANG N, SUN H, YANG P, LENG X Y, CHEN J Y, WAN G J, CHU P K. Surface characterization and blood compatibility of poly (ethylene terephthalate) modified by plasma surface grafting [J]. Surface and Coatings Technology, 2005, 196(1-3): 307-311. DOI: 10.1016/j.surfcoat.2004.08.161.
[94] HIGUCHI A, SHIRANO K, HARASHIMA M, YOON B O, HARA M, HATTORI M, IMAMURA K. Chemically modified polysulfone hollow fibers with vinylpyrrolidone having improved blood compatibility [J]. Biomaterials, 2002, 23(13): 2659-2666. DOI: 10.1016/S0142- 9612(01)00406-9.
(Edited by ZHENG Yu-tong)
中文导读
利用脉冲激光沉积和射频磁控溅射在Ti64基底上制备多功能磷锌矿纳米涂层于骨科植入物的比较研究
摘要:功能化种植体展示了骨科种植体的升级方法,旨在通过改进生物整合实现长期成功。多功能的生物陶瓷涂层由于其结合了生物植入物所必需的各种性能,如骨整合和抗菌特性,已成为传统涂层的有效替代品。本文利用脉冲激光沉积(PLD)和射频磁控溅射(RFMS)在Ti64基底上制备了薄磷锌矿涂层。使磷锌矿涂层在500 °C的真空中退火,并分别通过扫描电子显微镜(SEM)、X射线衍射(XRD)、原子力显微镜(AFM)、张力计、 荧光激活细胞排序(流式细胞仪)、模拟体液(SBF)浸泡试验和接触角测角仪研究表面形态、相组成、表面粗糙度、粘附强度、抗菌功效、磷灰石形成能力和表面润湿性能。结果表明,在Ti64基底上由PLD和RFMS两种新工艺路线合成的新一代多功能磷锌矿涂层是传统涂层的有效替代品。所沉积的磷锌矿涂层具有较强的骨整合能力和抗菌特性,保证了金属骨科种植体的整体稳定性。
关键词:磷锌矿;Ti64合金;脉冲激光沉积;磁控溅射;涂层;植入物
Received date: 2019-11-06; Accepted date: 2020-05-06
Corresponding author: Ashish DAS, PhD, Assistant Professor; Tel: +91-7061411736; E-mail: ashishdas.1110@gmail.com; ORCID: https://orcid.org/0000-0002-4099-1035
Abstract: Functionalized implants demonstrate an upgraded approach in orthopedic implants, aiming to achieve long term success through improved bio integration. Bioceramic coatings with multifunctionality have arisen as an effective substitute for conventional coatings, owing to their combination of various properties that are essential for bio-implants, such as osteointegration and antibacterial character. In the present study, thin hopeite coatings were produced by Pulsed laser deposition (PLD) and radio frequency magnetron sputtering (RFMS) on Ti64 substrates. The obtained hopeite coatings were annealed at 500 °C in ambient air and studied in terms of surface morphology, phase composition, surface roughness, adhesion strength, antibacterial efficacy, apatite forming ability, and surface wettability by scanning electron microscope (SEM), X-ray diffraction (XRD), atomic force microscope (AFM), tensometer, fluorescence-activated cell sorting (FACS), simulated body fluid (SBF) immersion test and contact angle goniometer, respectively. Furthermore, based on promising results obtained in the present work it can be summarized that the new generation multifunctional hopeite coating synthesized by two alternative new process routes of PLD and RFMS on Ti64 substrates, provides effective alternatives to conventional coatings, largely attributed to strong osteointegration and antibacterial character of deposited hopeite coating ensuring the overall stability of metallic orthopedic implants.
- Multifunctional hopeite nanocoating on Ti64 substrates by pulsed laser deposition and radio frequency magnetron sputtering for orthopedic implant applications: A comparative study
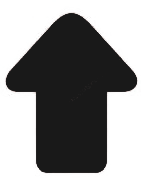