- Abstract:
- 1 Introduction▲
- 2 Experimental ▲
- 4 Discussion▲
- 5 Conclusions▲
- References
- Figure
- Fig.1 Microstructure of core zone with fine η phase (dark spot) in state of dispersed distribution
- Fig.2 Distribution maps of Ce(a) and O(b) on sinter skin[3]
- Fig.3 Back-scattered electron image of core area with WC+β+η three-phase structure without etching
- Fig.4 EDXS spectra of different zones in Fig.3: (a) Zone b; (b) Zone c; (c) Zone d
- Fig.5 TRS fractograph of alloy with easily indentified fracture source
- Fig.6 EDXS spectrum of fracture source area shown in Fig.5
J. Cent. South Univ. Technol. (2008) 15: 6-10
DOI: 10.1007/s11771-008-0002-2
Directional migration behavior of cerium during sintering process of mischmetal doped cemented carbide
ZHANG Li(张 立)1, CHEN Shu(陈 述)2, XIONG Xiang-jun(熊湘君)1,
YU Xian-wang(余贤旺)1, WANG Yuan-jie(王元杰)1
(1. State Key Laboratory of Powder Metallurgy, Central South University, Changsha 410083, China;
2. Changsha Mining and Metallurgy Research Institute, Changsha 410012, China)
Abstract:
Three observation methods were used to investigate the existing form and the behavior of rare earth during the sintering process of high activity mischmetal (RE, with lanthanum and cerium) doped WC-8%Co-0.048%RE(mass fraction) alloy with low carbon-containing level by scanning electron microscopy (SEM) and energy dispersive X-ray spectroscopy (EDXS), considering the fact that the addition amount of rare earth in the alloy is very minute. The directional migration process and mechanism of cerium were discussed. First, the sinter skin (surface) is observed. It is shown that there exists a dispersedly distributed cerium containing oxide on the sinter skin, and lanthanum in these cerium enrichment positions is very minute. Secondly, the polished section is observed, and lanthanum containing phase/micro-zone in the alloy is identified. Finally, based on the fact that the fracture of cemented carbide is resulted from the heterogeneous phase or other defects within the microstructure, the fracture surface is observed and cerium containing phase/micro-zone in the fracture source approximately 260 μm from the surface is identified. These combined observations reveal adequately the fact that lanthanum and cerium get separated and cerium predominantly migrates towards the surface during the sintering process.
Key words:
cemented carbide; rare earth; surface segregation; directional migration; liquid phase sintering;
1 Introduction
Recently, an enrichment phenomenon of rare earth on the sinter skin of rare earth doped cemented carbide has been reported by ZHANG et al[1-3]. It is shown that the behavior of rare earth in high-activity mischmetal doped cemented carbide varies with the carbon-containing level in the alloy and the size of the sample (size effect).
As part of series study of acting mechanism and behavior of rare earth in cemented carbide, the phenomena of the separation of lanthanum and cerium and the directional migration of cerium during the sintering process and the existing form of rare earth in the alloy were studied in this work. The confirmation of the directional migration ability of rare earth in the alloy and the study of the migration mechanism are not only of scientific value, but also of very important reference value for the understanding of the performance improvement of rare earth doped cemented carbide, the electron emission performance of rare earth doped molybdenum cathode material.
2 Experimental
Tungsten carbide powder, cobalt powder and
mischmetal-cobalt pre-alloyed powder with RECo5 as main phase and oxygen content of 0.36%(mass fraction) according to the composition of WC-8%Co- 0.048%RE (mass fraction) were ball-milled for 72 h. The mass fractions of lanthanum and cerium in mischmetal are 30.15% and 52.17%, respectively. Sintering was carried out in a conventional vacuum-sintering furnace at 1 440 ℃ for 60 min. A dual phase (DP) structure[4-7] is observed. From the cross sections of the samples with size of 5 mm×5 mm, WC+β two-phase structure is observed in the outer layer of approximatly 0.5 mm in thickness and WC+β+η three-phase structure is observed in the core, as shown in Fig.1. A dispersedly distributed cerium containing oxide on the sinter skin is identified, as shown in Fig.2. However, in the cerium enrichment areas, the amount of lanthanum is very minute. Because lanthanum and cerium are in an unlimited solid solution state within mischmetal, the location of lanthanum in the alloy must attract a lot of attention.
Based on the fact that cerium is in a highly enrichment state, it can be deduced that lanthanum can also be in an enrichment state in the alloy and in the mean time, it is possible that there exists cerium enrichment micro zone near the sinter skin. Considering the facts that the addition amount of mischmetal is very minute, and cerium is already in high enrichment state on the sinter skin, as well as the possible enriched lanthanum or cerium containing phase or zone in the alloy is in the state of stochastic distribution, two different methods were used in the search of the lanthanum or cerium in the alloy. First, the polished cross section was observed. Then, based on the fact that the fracture of cemented carbide is caused by the heterogeneous phase or other defects within the microstructure, the fracture surface was observed.
Fig.1 Microstructure of core zone with fine η phase (dark spot) in state of dispersed distribution
Fig.2 Distribution maps of Ce(a) and O(b) on sinter skin[3]
3 Results3.1 Observation and analysis of microstructure
The microstructure in the core of the polished section is shown in Fig.3. EDXS spectra of the bright triangle micro zone with arrow b (zone b), the dark micro zones with arrows c and d (zones c and d) in Fig.3 are given in Fig.4. It is known from Fig.4 that there are
Fig.3 Back-scattered electron image of core area with WC+β+η three-phase structure without etching
Fig.4 EDXS spectra of different zones in Fig.3: (a) Zone b; (b) Zone c; (c) Zone d
lanthanum and trace cerium in zone b. However, only lanthanum is detected in zones c and d. Oxygen is not detected in these areas. Based on the results from Fig.2 and Fig.4, it can be concluded that nearly all the cerium atoms in the alloy migrate to the sinter skin to combine with the oxygen from the sintering atmosphere.
It can be deduced from the color and the shape that the triangle phase ahead of arrow b indicated in Fig.3 is tungsten carbide. However, because the basic compositions of both η phase and the binder of WC-Co cemented carbide are the same, i.e. cobalt, tungsten and carbon, the average atomic number of components in η phase and the binder are the same, it is difficult to distinguish η phase from the binder from the back-scattered electron (BSE) image when the η phase grains are fine in size without inlaid WC grains. Therefore, zones c and d can be either η phase or the binder zone.
3.2 Observation and analysis of fracture surface
In the case of decarbonization of WC-Co cemented carbide, it is very common that the third phase, i.e. η phase in the alloy is very coarse in size with inlaid WC grains and in a state of heterogeneous distribution. Usually, the transverse rupture strength (TRS) of this kind of alloy is less than 1.5 GPa. Nevertheless, it is shown that the average value of TRS of five samples with structure shown in Fig.1 and dimensions of 5 mm×5 mm×35 mm is 2.58 GPa. Fractograph of the sample with TRS of 2.699 GPa is shown in Fig.5. EDXS spectrum of the position of fracture source in the center of a square marked in Fig.5 is shown in Fig.6. It is known from Fig.6 that only cerium is detected in the zone near the sinter skin and legible oxygen peak does not exist in this zone, which indicates that the migration of cerium towards the sinter skin overtakes one of oxygen towards the inner and the existing forms of rare earth in the alloy and on the sinter skin are quite different. Cerium not only exists on the sinter skin as indicated in Fig.2, but also exists in the zone near the sinter skin in another form.
Fig.5 TRS fractograph of alloy with easily indentified fracture source
Fig.6 EDXS spectrum of fracture source area shown in Fig.5
4 Discussion
From SEM and EDXS observation and analysis results of the sinter skins, cross section and fracture surface, coupled with the fact that the addition amount of mischmetal is very minute, it is known that during the sintering process, cerium gets separated with lanthanum in mischmetal, and enriched on the sinter skin.
The separation of lanthanum and cerium during the sintering process under vacuum indicates that there is a competition mechanism between lanthanum and cerium to combine with the limited oxygen in the sintering atmosphere. Although this competition mechanism can be explained from thermodynamic point of view, by the diagram of standard-state Gibbs free energies of formation of lanthanum and cerium oxides, i.e. La2O3, Ce2O3 and CeO2 per unit volume (ΔfGV?) against temperatures[8], it still remains difficult in understanding the fact of the directional migration of cerium towards the sinter skin during the sintering process and the co-existed fact that there are rare earth atoms in tungsten carbide and the binder phase or η phase zones, for it is a well accepted fact that the difference in the atomic radius between cobalt and cerium is 46.92%, cobalt and lanthanum 50.16%, tungsten and cerium 40.31%, tungsten and lanthanum 44.38%. Generally speaking, it is thermodynamically impossible for lanthanum and cerium atoms to take the position of cobalt atom and/or tungsten atom, thus forming solid solution in the binder, tungsten carbide and η phase, which should result in dramatic increase in free energy of the alloy system.
Nevertheless, due to the special electronic configurations of rare earth atoms, a strong interaction among rare earth atoms and nonmetallic atoms whose values of electronegative parameter are much bigger than those of rare earth atoms can result in the polarization or ionization of rare earth atoms and thus result in the reduction in atomic radii of rare earth atoms[9]. In fact, JI et al[10] have proved the solid solution phenomenon of rare earth in steel by the means of internal friction research.
As is known, the lower the carbon-containing level in WC-Co cemented carbide, the much higher the solubility of tungsten in the binder. Taking WC-25Co cemented carbide as an example, when the total carbon-content in the alloy decreases from 4.52% to 4.25%(mass fraction), tungsten-content in the binder increases from 2.6% to 20.2%[11]. When the carbon-containing level in the alloy is too low, η phase will form according to the reaction[12]:
3WC+3Co(l)→W3Co3C(M6C)+2C (1)
The formation of η phase results in the instantaneous existence of free carbon atoms with high energy contributed by the enhanced movement of atoms at the boundary between tungsten carbide and the liquid binder caused by the increasing dissolubility of tungsten in the binder. Therefore, it is most probable that the interaction among the high energy carbon atom, with value of electronegative parameter of 2.50, cerium and lanthanum atoms with values of electronegative parameter of 1.12 and 1.10, respectively should result in the polarization of cerium and lanthanum atoms, and thus the formation of solid solution phase containing lanthanum and/or cerium. On the other hand, the interaction among the high energy carbon atom, cerium atom and lanthanum atom can obstruct the diffusion of the excessive carbon atom, and thus restrain the growth of η phase and facilitates the nucleation of η phase, as indicated in Fig.1.
The reasonable way to understand the fact that there are lanthanum and trace cerium atoms in the zone of tungsten carbide is that there is polarization phenomenon of lanthanum and cerium atoms during the sintering process. Under the condition of the polarization of cerium and thus the reduced atomic radius, it becomes possible for the migration of cerium even through tungsten carbide towards the sinter skin to combine with oxygen during the sintering process. If the polarization of lanthanum and cerium atoms makes the atomic radii comparable with that of tungsten or cobalt, from thermodynamical point of view, lanthanum or cerium can exist in the form of solid solution when the addition amount is very minute, because the formation of a new phase can result in remarked increase of phase interface energy.
From La-Co and Ce-Co phase diagrams[13-14], it can be deduced that the melting point of mischmetal-cobalt alloy with RECo5 as its main phase composition is around 1 200 ℃. It is the low melting point of RE-Co alloy that the eutectic temperature of WC-Co alloy doped with high activity rare earth can be lowered[15]. Because of the increased migration channels and increased energy of the atoms, the existence of liquid phase facilitates the diffusion and migration of atoms. Therefore, it can be deduced that the separation of lanthanum and cerium and the directional migration of cerium atom from the inner of cemented carbide to the sinter skin should take place at the stage of liquid phase sintering and the directional migration of cerium atom should be along the fast channels through the liquid binder phase, boundaries between the liquid binder and tungsten carbide, even across the tungsten carbide phase. Nevertheless, from the fact that there are lanthanum and trace cerium in WC zone, it can be deduced that when the directional migration of cerium atom passes across the tungsten carbide phase, the solid phase, i.e. tungsten carbide can act as a trap.
5 Conclusions
1) There is a competition mechanism between lanthanum and cerium to combine with the limited oxygen in the sintering atmosphere. As a result, lanthanum remains in the alloy, while cerium predominantly migrates towards the surface. Because the migration of cerium towards the surface overtakes that of oxygen towards the inner, the existing forms of rare earth in the alloy and on the sinter skin are quite different.
2) The confirmation of atom polarization and directional migration ability of rare earth, as well as the study of the migration mechanism provide a clue to understand the performance improvement of rare earth doped cemented carbide, the electron emission performance of rare earth doped molybdenum cathode material.
3) The interaction among carbon, cerium and lanthanum atoms facilitates the formation of fine η phase, which provides an effective method to control the microstructure of functionally graded cemented carbide with dual phase structure.
References[1] ZHANG Li, SCHUBERT W D, HUANG Bai-yun, WU En-xi. Surface observation of rare earth doped cemented carbide[J]. Journal of Central South University of Technology: Science and Technology, 2004, 35(5): 742-747. (in Chinese)
[2] ZHANG Li, SCHUBERT W D, CHEN Shu, ZHANG Chuan-fu, HUANG Bai-yun. Rare earth enrichment phenomenon during the sintering process of grainy hardmetal[J]. Materials Science and Engineering A, 2004, 384 (1/2): 395-401.
[3] ZHANG Li, CHEN Shu, XIONG Xiang-jun, HUANG Bai-yun, ZHANG Chuan-fu. Cerium enrichment on sinter skin of mischmetal doped WC-Co cemented carbide[J]. Journal of Central South University of Technology, 2005, 12(s1): 5-8.
[4] ZHANG Li, HUANG Bai-yun, WU En-xi. Research on dual phase cemented carbides[J]. Materials Review, 2000, 14(12): 17-19. (in Chinese)
[5] ZHANG Li, CHEN Shu, XIONG Xiang-jun, HUANG Bai-yun. Microstructure and Vickers hardness of functionally graded WC-Co cemented carbide with dual phase structure[J]. The Chinese Journal of Nonferrous Metals, 2005, 15(8): 1194-1199. (in Chinese)
[6] ZHANG Li, CHEN Shu, XIONG Xian-jun, HE Yue-hui, HUANG Bai-yun, ZHANG Chuan-fu. Phase composition, phase transition and phase structure stability of functionally graded cemented carbide with dual phase structure[J]. Journal of Central South University of Technology, 2007, 14(2): 149-152.
[7] LIU Yong, WANG Hai-bin, YANG Jian-gao, HE Yue-hui, LONG Zheng-yi. Relationship between structure and properties of graded cemented carbide[J]. Materials Science and Engineering of Powder Metallurgy, 2005, 10(6): 356-360. (in Chinese)
[8] ZHANG Li, CHEN Shu, SCHUBERT W D, HUANG Bai-yun. Thermodynamic analysis on the phenomenon of the enrichment of Ce on the sinter skin during the sintering of mischmetal doped cemented carbide[J]. Cemented Carbide, 2004, 21(2): 70-76. (in Chinese)
[9] JI Zhe-sheng, XIA Li-fang, WAN Hai-bo, WU Yun-qi, ZHAO Mi, CHENG Xiao-hui. Phase composition of initial rare earth-B-Al permeation layer[J]. The Chinese Journal of Nonferrous Metals, 1998, 8(s1): 66-69. (in Chinese)
[10] JI Jing-wen, CHE Yun-yi, LIU Ai-sheng, LU Xiao-li, YUO Miao. Research and theory in internal friction of alloying of rear earth in iron and steel[J]. Journal of the Chinese Rare Earth Society, 1996, 14(4): 350-359. (in Chinese)
[11] KOLASKA H, HIRSCHFELD D, GREWE H. Powder metallurgy of hardmetals[M]. Vol.9. Shrewsbury: EPMA, 1995: 22.
[12] SARIN V K. Morphology of Eta phase in cemented WC-Co alloys[J]. Modern Development in Powder Metallurgy, 1977, 10: 553-565.
[13] PETROVA L A, EFFENBERG G. Red book-phase diagrams of Metallic systems[M]. Vol.35. Stuttgart: Material Science International Services GmbH, 1990: 137.
[14] BAKER H, OKAMOTO H. ASM handbook: Alloy phase diagrams[M]. Vol.3. Ohio: American Society for Metals, 1992: 151-153.
[15] XU Chong-hai, AI Xing, HUANG Chuan-zhen. Research and development of rare-earth cemented carbides[J]. International Journal of Refractory Metals and Hard Materials, 2001, 19(3): 159-168.
(Edited by CHEN Wei-ping)
Foundation item: Project(50574104) supported by the National Natural Science Foundation of China
Received date: 2007-06-19; Accepted date: 2007-08-22
Corresponding author: ZHANG Li, PhD, Professor; Tel: +86-731-8876424; E-mail: zhangli@mail.csu.edu.cn
- Directional migration behavior of cerium during sintering process of mischmetal doped cemented carbide
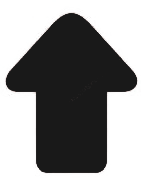