- Abstract:
- 1 Introduction▲
- 2 Experimental▲
- 3 Overall electrode react...▲
- 4 Electrode reaction cour...▲
- 5 Conclusions▲
- References
- Figure
- Fig.1 shows the relationship between lg Jh and 1/T at the same overpotential based on the relationship between apparent activation energy and temperature. The slope of the line in Fig.1 is –2 032.76, and the calculated apparent activation energy is 38.93 kJ/mol, slightly less than 40 kJ/mol, which indicates that the influence of the preceding reaction on electrochemical reaction is not obvious. The reason is that on the one hand the concentration of Zn(OH)42- is higher, on the other hand the speed of preceding reaction is fast. So it can be concluded that the electrochemical reaction is the rate-determining step during Zn depositing in alkaline zincate solution.
- Fig.2 Relationship between Jτ1/2 and J of zinc depositing in alkaline solution
- Fig.3 V-t curve of chronopotentiometry
- Fig.4 Cyclic voltammetry curve of electrolyte at scan rate of 0.02 V/s and 25 ℃
- Fig.5 Relationship between lg J0 and of zinc depositing in alkaline solution
- Fig.6 Alternating current impedance diagram of electrode reaction under different potentials at 100 kHz, 30℃ in swing range of sine wave of 5 mV
J. Cent. South Univ. Technol. (2007)01-0037-05
DOI: 10.1007/s11771-007-0008-1
Mechanism of zinc electroplating in alkaline zincate solution
PENG Wen-jie(彭文杰), WANG Yun-yan(王云燕)
(School of Metallurgical Science and Engineering, Central South University, Changsha 410083, China)
Abstract:
The cathodic deposition properties and mechanism of Zn in alkaline zincate solution were studied by electrochemical techniques. The results show that Zn2+ exists in the alkaline solution in the form of Zn(OH)42-. The apparent activation energy of the electrode reaction is 38.93 kJ/mol, which indicates that the discharge of Zn(OH)42- on cathode is controlled by electrochemical polarization, and accompanied by a preceding chemical reaction. The diffusion coefficient of Zn(OH)42- is 2.452×10-6 cm2/s. Zn(OH)2 is the species directly discharged on the cathode surface. Based on the above results the mechanism of zinc electroplating in alkaline zincate solution was put forward. The discharged species is Zn(OH)2 formed from the preceding chemical reaction, which becomes Zn(OH)ad when gaining one electron, and then gaining the second electron to become Zn. The first electron gaining step is rate determining one.
Key words:
Zn electroplating; mechanism; alkaline; zincate;
1 Introduction
The electrochemistry of metal deposition and dissolution has been an applied science, and only in recent years has some fundamental knowledge been accumulated in this field[1-6].
The corrosion resistance of steel substrate was improved greatly with the development of electroplated zinc coatings. Among all kinds of electroplating systems, zinc coatings electroplated in alkaline zincate solution have many good properties, such as excellent corrosion resistance, good ductibility, well painted characteristics, low hydrogen embrittlement, small internal stress, and so on, and alkaline zincate system with the strongpoints of simple components, well spreading and covering power, being propitious to electroplate complicated accessory, less corrosion to equipment, lower cost and so forth attract more attention throughout the world. However, the studies and applications have mainly focused on the electroplating techniques, little on the deposition mechanism[7-11].
Some fundamental works have been reported on the zinc/alkaline zincate system, but the experimental results and conclusions of the different investigators are contradictory. Several mechanisms of zinc electrode in the alkaline solution, such as alkaline batteries, were proposed. The first typical one proposed by BERNNER[12] is as follows: the discharged species is Zn(OH)2, which becomes Zn(OH)2-ad when gaining two electrons once, and then loses OH- to become Zn, that is, Zn(OH)42-→ Zn(OH)2+2OH-; Zn(OH)2+2e→Zn(OH)2-ad; Zn(OH)2-ad→Zn+OH-. In this mechanism, the second reaction is rate determining step. The second typical mechanism was proposed by BOCKRIS et al[13], it is considered that Zn(OH)3- is the discharged species, gaining electrons step by step, meanwhile Zn(OH)ad is formed through the chemical transforming reaction, that is, Zn(OH)42-→Zn(OH)3-+OH-, Zn(OH)3-+e→Zn(OH)2-+OH-, Zn(OH)2-→Zn(OH)ad+OH-, and Zn(OH)ad+e→Zn+OH-. In this mechanism, the first electron gaining reaction is the rate determining step.
So in this study, the deposition mechanism of Zn in alkaline zincate electrolytes was investigated using a variety of electrochemical techniques, and then the cathodic reaction course of Zn was proposed, which will be a guidance for zinc electroplating for corrosion resistance of steel substrate.
2 Experimental
Three electrode system with “H” style cell using sand core glass septum as electrobath, working electrode was the platinum electrode with the area of 0.984 mm2 in order to eliminate the effect of hydrogen evolution in alkaline solution, a platinum piece with great area compared with working electrode was used as counter electrode, and Hg/HgO electrode dipped in 1mol/L NaOH solution as reference electrode. Salt bridge with Luggin capillary was used to eliminate the border potential between different solutions and to decrease the resistance of solution. The surface of the sample was polished with abrasive paper, dipped successively in acetone and washed by redistilled water before immersion in the test solution. Before commencing polarization measurements, the solution was purged by a stream of purified N2 for at least 15 min in order to remove the dissolved oxygen. The cell was maintained in a thermostated water bath to keep a constant temperature of 28 ℃, The scan rate of steady-state polarization was controlled at 1 mV/s and measured by CHI660A electro-chemical workstation monitored by computer.
The electrolyte was composed of 18 g/L ZnO and 180 g/L NaOH dissolved in redistilled water, which were all analytical reagents.
3 Overall electrode reaction
Metal Zn is formed through cathodic deposition of the bivalent zinc ion in electrolyte, which indicates that electrode reaction gains two electrons. In addition, the predominant solution species is Zn(OH)42-. So the overall electrode reaction can be written as follows:
Zn(OH)42-+2e=Zn+4OH- (1)
4 Electrode reaction course
4.1 Characteristic of rate determining step
The result of cyclic voltammetry indicates that the cathodic discharge of Zn(OH)42- is irreversible, whether it is controlled by electrochemical polarization or the concentration polarization should be testified further. Apparent activation energy of electrode reaction is efficient[14]. The apparent activation energy is 12-16 kJ/mol when the electrode reaction is controlled by species diffusion step; while that is more than 40 kJ/mol when the electrochemical reaction is the rate determining step. However the apparent activation energy is similar to that of diffusion process if there exists preceding reaction of chemical transforming, and its value is related to the speed of the preceding reaction and the concen- tration of the mainly existing ions.
Fig.1 shows the relationship between lg Jh and 1/T at the same overpotential based on the relationship between apparent activation energy and temperature. The slope of the line in Fig.1 is –2 032.76, and the calculated apparent activation energy is 38.93 kJ/mol, slightly less than 40 kJ/mol, which indicates that the influence of the preceding reaction on electrochemical reaction is not obvious. The reason is that on the one hand the concentration of Zn(OH)42- is higher, on the other hand the speed of preceding reaction is fast. So it can be concluded that the electrochemical reaction is the rate-determining step during Zn depositing in alkaline zincate solution.
Fig. 1 Relationship between current density and temperature of zinc depositing in alkaline solution at overpotential of 0.035 V
4.2 Preceding chemical reaction
In order to find out the reduction course of metal complex, the form metal ion existing in the solution participates directly in electrode reaction should be confirmed firstly. Chronopotential experiment of electrolyte was performed at different current densities for estimating whether the preceding chemical transforming reaction undergoes or not before the discharge of Zn(OH)42-.
The experimental data abide with the following equation if a preceding reaction exists[14],
(2)
where K1 and K-1 are respectively the rate constants of positive and reverse reaction, K=K1/K-1, D is the diffusion coefficient, r=aO0+aR0, aO0 is the initial activity of reactant, aR0 is the initial activity of the product, A is the electrode area, and is the transition time. The relationship between Jτ1/2 and J is linear when J is low, whereas Jτ1/2 is independent of J when J is high.
Fig.2 shows the relationship between Jτ1/2 and J of zinc depositing in alkaline solution. There exists a linear relationship between Jτ1/2 and J when J is low, and the slope is negative; while Jτ1/2 is independent of J when J is high enough, the line is approximately parallel to abscissa, the curve increases slightly when current density is high because of the influence of double layer charging. The results agree with the theoretical equation, which indicates that preceding chemical transforming reaction occurs before the discharge of Zn(OH)42-.
Fig.2 Relationship between Jτ1/2 and J of zinc depositing in alkaline solution
4.3 Charges transfer step by step
4.3.1 Chronopotentiometry experiment
Potential-time curve of chronopotentiometry at I=80 μA is shown in Fig.3. It is evident that two parallel charge transfer processes corresponding to two transition times take place before hydrogen evolution largely, the transition times are τ1=24 s and τ2=12 s, which indicate that zinc ions discharge by two steps[14].
Fig.3 V-t curve of chronopotentiometry
4.3.2 Diffusion coefficient
The relationship between the peak current density and the scan rate of cyclic voltammetry curve of irreversible electrode reaction[14] is as follows:
(3)
where D0 is the diffusion coefficient and υ is the scan rate, R is the gas constant, 8.314 J/(mol?K), n is the electron number participating in the reaction, F=96 485 C/mol, α is the transfer coefficient. Fig.4 shows the cyclic voltammetry curve of the electrolyte measured at 25 ℃ and the scan rate of 0.02 V/s. The cathodic peak current density Jp is 246.8 μA/dm2, the cathodic apparent transfer coefficient measured by steady-state polarization curve is 0.519 3, D0=2.452×10-6 cm2/s (commonly 10-5cm2/s) is calculated according to Eqn.(3), which is less because the size of Zn(OH)42- is bigger than simple specie.
Fig.4 Cyclic voltammetry curve of electrolyte at scan rate of 0.02 V/s and 25 ℃
4.3.3 Theoretical calculation of transition time
τ1 and τ2 are the transition times of the first and second wave, corresponding to the discharge of formed from preceding chemical reaction and the reaction of
respectively in the V-t curve of chronopotentiometry, which can be expressed as:
Ox+e→O2 (4)
O2+e→R (5)
Suppose that
τ2=+
(6)
O2, which does not exist originally, is only the product of deoxidization of Ox in the electrode reaction course. So O2 partly stays on the electrode surface and another part diffuses into the solution. According to the current reverse technique of soluble product[14], 2/3 of O2 is entered into the solution through diffusion, thus the time that O2 stayed on electrode surface is deoxidized further is . The experimental results indicate that τ 1=24 s and τ 2=12 s, and τ′=8 s, τ″=τ 2-τ′=4 s.
It can be considered that τ′ is the time of the reaction of Ox on the electrode surface diffused from the solution owing to the concentration grades within τ′. Thereby the concentration grads on electrode surface is
Based on
(7)
The reaction current density of this part of Ox is
(8)
That is
=Jτ″ (9)
Thereby
(10)
=3.72 s≈4 s can be calculated according to Eqn.(10) and A=0.984 mm2, D0=2.452×10-6 cm2/s,
=0.178 mol/L, J=80 μA/dm2, which are consistent with the experimental results.
4.4 Form of preceding reaction and discharge ion
It has been proven that the preceding chemical reaction undergoes before the discharge of Zn(OH)42-, so the form of the preceding reaction should be determined. Suppose the preceding reaction is
Zn(OH)42-→+(4-δ)OH- (11)
where δ is the number of complex which discharges directly. The concrete form of the preceding reaction can be confirmed completely if δ is measured.
On condition of a preceding reaction existing, half limit diffusion and a great deal of sustaining electrolyte existing, the relationship between the exchange current density and the complex reagent activity can be described by the following differential equation[14],
(12)
where J0 is the exchange current density,A/dm2, μis the number of complex mainly existing, ax is the activity of the complex reagent in mol/mL, is the cathodic
transfer coefficient, is the activity of metal
complex, aM is the activity of metal ion. So
lg J0=B+[δ-(1-)μ]lg ax (13)
where B is a constant. The above equation shows that there is a linear relationship between lg J0 and lg ax, and the slope is δ-(1-)μ, from which δ can be calculated.
Tafel curves were obtained at different OH- activities, the relationship between lg J0 and is found (see Fig.5), and the slope is 0.318 0.
=0.519 3 is calculated by steady-state polarization curves. Forμ= 4, δ=2.240 8≈2 can be calculated from the equation δ-(1-
)μ=0.318 0. Therefore Zn(OH)2 is the complex ion discharging directly on the cathode, hence the preceding reaction is
Zn(OH)42-==Zn(OH)2+2OH- (14)
Fig.5 Relationship between lg J0 and of zinc depositing in alkaline solution
4.5 Alternating current impedance
From the above results it can be seen that Zn(OH)ad forms in the second step. This kind of complex ion exists on electrode surface in the form of active adsorbent state because Zn+ is unstable. In order to confirm this standpoint further investigation and testification should be carried through. Fig.6 shows the alternating current impedance diagram of Zn(OH)42- at different potentials in alkaline zincate electrolyte.
Fig.6 Alternating current impedance diagram of electrode reaction under different potentials at 100 kHz, 30℃ in swing range of sine wave of 5 mVPotential(vs Hg/HgO): 1-1.45 V; 2-1.50 V
It can be seen from Fig.6 that the alternating current impedance diagram of electrode reaction is composed of two semicircles lying in the first and the forth quadrants respectively. Moreover, the radius of the first semicircle decreases and that of the second one decreases more rapidly when the cathodic potential moves positively, which indicates that the discharge of Zn(OH)42- on cathode is controlled by electrochemical polarization, two charges transfer step by step; there exists adsorbent intermediate species with electro-activity during the electrode reaction.
4.6 Mechanism of electrode reaction
It can be concluded from the above experiments and analysis that the discharge of Zn(OH)42- on electrode is controlled by electrochemical polarization, Zn(OH)2 forms through preceding reaction before the discharge of Zn(OH)42-, two charges transfer step by step, while commonly the first electron received is slow for electrode reaction with many electrons, this step is rate-determining step.
Therefore, the mechanism of Zn(OH)42- depositing in alkaline zincate solution is determined as
Zn(OH)42-==Zn(OH)2+2OH- (15)
Zn(OH)2+e→Zn(OH)ad+OH- (16)
Zn(OH)ad+e==Zn+OH- (17)
Thereinto, reaction (16) is the rate determining step of the electrode reactions. This mechanism is in agreement with the experimental facts.
5 Conclusions
1) The apparent activation energy of electrode reaction of Zn electrodating is 38.93 kJ/mol, which indicates that the discharge of Zn(OH)42- at electrode is controlled by electrochemical polarization.
2) There is a linear relationship between Jτ1/2 and J with negative slope when the current density is low; whereas Jτ1/2 is approximately parallel to abscissa, independent of J when J is high enough, which indicates that the preceding chemical transforming reaction occurs before the discharge of Zn(OH)42-. Zinc ions discharge by two steps and the transition times are τ1=24 s and τ2=12 s respectively. τ″=3.72 s is calculated, which is consistent with the experiment result (4 s).
3) The diffusion coefficient of Zn(OH)42- is 2.452×10-6 cm2/s, Zn(OH)2 is the complex ion discharging on electrode surface directly.
4) The discharged species is Zn(OH)2 formed from the preceding chemical reaction, which becomes Zn(OH)ad when gaining one electron, and then gaining the second electron to become Zn. The first electron gaining step is rate determining one.
References
[1] YANG C Q, LONG Z L, ZHOU Y C. A sulphate bath for the preparation of Zn-Fe alloy coatings[J]. Transactions of the Institute of Metal Finishing, 2002, 80(5): 161-163.
[2] TREJO G, ORTEQA R, MEAS Y, et al. Effect of benzylideneacetone on the electrodeposition mechanism of Zn-Co alloy[J]. Journal of Applied Electrochemistry, 2003, 33(5): 373-379.
[3] ORDINE A P, DIAZ S L, MARQARIT I C P, et al. Zn-Ni and Zn-Fe alloy deposits modified by P incorporation: anticorrosion properties[J]. Electrochimica Acta, 2004, 49(17/18): 2815-2823.
[4] YANG C Q, ZHOU Y C, LONG Z L. Electrodeposition and physico-chemical properties of Zn-Fe alloy coatings from sulfate solution[J]. Journal of Materials Science Letters, 2002, 21(21): 1677-1680.
[5] LONG Z L, ZHOU Y C, XIAO L. Characterization of black chromate conversion coating on the electrodeposited zinc-iron alloy[J]. Applied Surface Science, 2003, 218(1/4): 123-136.
[6] IQARASHI K, ARAMATA A, TAQUCHI S. Underpotential deposition of zinc ions and specific adsorption of hydroxyl species at Pt(Ⅲ) in alkaline solutions[J]. Electrochimica Acta, 2001, 46(12): 1773-1781.
[7] YU jing-xian, WANG Lei, SU Lei, et al. Temperature effects on the electrodeposition of zinc[J]. Journal of the Electrochemical Society, 2003, 150(1): C19-C23.
[8] WANG Yun-yan, PENG Wen-jie, CHAI Li-yuan, et al. Electrochemical behaviors of Zn-Fe alloy and Zn-Fe-TiO2 composite electrodeposition[J]. Journal of Central South University of Technology, 2003, 10(3): 183-189.
[9] de WIT K, de COOMAN B C, de BOECK A. Reassessment of the Zn-Fe phases in electrodeposited Zn-Fe layers[J]. Materials Research and Advanced Techniques, 2000, 91(5): 421-428.
[10] Abd-EI REHIM S S, EMAD M, KHALED M, et al. Electrodeposition of Zn-Fe alloys from aqueous acetate baths[J]. Transactions of the Institute of Metal Finishing, 2001, 79(3): 95-98.
[11] BAJAT J B, MISKOVIC-STANKOVIC V B, KACAREVIC- POPOVIC Z. The influence of steel surface modification by electrodeposited Zn-Fe alloys on the protective behaviour of an epoxy coating[J]. Progress in Organic Coatings, 2003, 47(1): 49-54.
[12] BERNNER A. Electrodeposition of Alloys[M]. New York and London: Academic Press, 1963: 222-235.
[13] BOCKRIS J O’M, NAGY Z, DAMJANOVIC A. On the deposition and dissolution of zinc in alkaline solutions[J]. Journal of the Electrochemical Society, 1972, 119(3): 285-295.
[14] SHU Yu-de, CHEN Bai-zhen. Investigating Methods of Metallurgical Electrochemistry[M]. Changsha: Central South University of Technology Press, 1990: 159-277. (in Chinese)
Foundation item: Project(50274073) supported by the National Natural Science Foundation of China
Received date: 2006-05-08; Accepted date: 2006-06-23
Corresponding author: WANG Yun-yan, PhD; Tel: +86-731-8830875; E-mail: wyy@mail.csu.edu.cn
- Mechanism of zinc electroplating in alkaline zincate solution
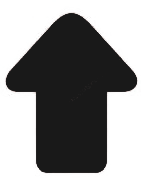