- Abstract:
- 1 Introduction▲
- 2 Solution-processed...▲
- 3 Solution-processed...▲
- 4 ETL and HTL for so...▲
- 5 HTL and ETL for so...▲
- 6 Solution-processed...▲
- 7 Conclusions and ou...▲
- References
- Figure
- Figure 1 Schematic illustration of PSCs with conventional mesoporous structure (a), conventional planar structure (b), and inverted planar structure (c)
- Figure 2 SEM images of perovskite films fabricated via one-step (a) and two-step (b) sequential deposition on FTO substrate, (c) J–V curves for a best-performing cell measured at a simulated AM 1.5G solar irradiation of 96.4 mW/cm2 (solid line) and in the dark (dashed line) [26] (Copyright 2013 Nature)
- Figure 3 Schematic of fully printable carbon-electrode meso-PSCs (a) (The mesoporous layers of TiO2 and ZrO2 have a thickness of ~1 and 2 mm, respectively, and are deposited on a FTO-covered glass sheet. They are infiltrated with perovskite by drop-casting from solution); (b) J-V curves under simulated AM 1.5 solar irradiation at an intensity of 100 mW/cm2 measured at room temperature; (c) and (d) TEM images of MAPbI3 and (5-AVA)x(MA)1-xPbI3 [28] (Copyright 2016 Science)
- Figure 4 Cross-sectional SEM image of PSCs (a); J-V curves of PSCs with (red) and without (black) Li doping (b); doped mp-TiO2 layer for the O 1s peaks (c) and the undoped control (d); Ti 3s and Li 1s peaks slightly visible at 55 eV for Li-doped (e) and the signal for the undoped TiO2, which reveals the absence of the peaks related to Ti 3s (dashed line at 61.5 eV) and Li 1s, dashed line at 54.9 eV (f); Charge transport lifetime as function of the Jsc prepared with (red) and without (black) Li-doped TiO2(g); Charge extracted at open circuit as function of the voltage for no treated samples (black) and Li-doped samples (red) [33] (h) (Copyright 2016 Nature)
- Figure 5 Schematic illustrating charge transfer and charge transport in a perovskite-sensitized TiO2 solar cell (left) and a noninjecting Al2O3-based solar cell (right) (a); J-V characteristics for Al2O3-based cells (red solid trace crosses for high efficiency of Al2O3-based cells, red dashed line with crosses for high Voc of Al2O3-based cells, black trace with circles for a perovskite-sensitized TiO2 solar cell, purple trace with squares for a planar-junction diode with structure FTO/compact TiO2/MAPbI2Cl/Spiro-OMeTAD/Ag) (b); PIA spectra of mp-TiO2 films (black circles) and Al2O3 films (red crosses) coated with perovskite with (solid lines) and without (dashed lines) Spiro-OMeTAD hole transporter (c);Charge transport lifetime determined by small-perturbation transient photocurrent decay measurement of perovskite-sensitized TiO2 cells (black circles) and Al2O3 cells (red crosses), both with lines to aid the eye (Inset shows normalized photocurrent transient for Al2O3 cells (red trace with crosses) and TiO2 cells (black trace with circles)) (d) [38] (Copyright 2012 Science)
- Figure 6 Schematic of interdiffusion deposition (a); SEM images of PbI2 film (b) and annealed MAPbI3 film deposited by an interdiffusion deposition (c) and one-step deposition (d) [53] (Copyright 2014 RSC)
- Figure 7 Schematic illustration of doctor-blade coating (a) and slot-die coating (b) [18] (Copyright 2019 Wiley)
- Figure 8 Schematics of spray coating (a) [51] and micro-gravure printing (b) [74] (Copyright 2019 RSC)
- Figure 9 Schematics illustrating representative post-growth treatments for perovskite films, i.e., Ostwald ripening, solvent annealing and methylamine gas treatment [19] (Copyright 2018 Nature)
- Figure 10 Top-view SEM images of perovskite films obtained by two-step spin coating using different ratios of ACN in DMF (a) [44] (Copyright 2016 Wiley); Schematic of the ligand exchange process and the proposed nucleation and growth routes of perovskite crystal films (b) (The assisted formation of lead-halide octahedrons in the vicinity of an MAI-capped PbS nanoparticle [45], Copyright 2016 RSC); Current density-voltage characteristics of devices with ITO/perovskite/Au configuration utilized for estimating the defect density in perovskite films (c) [98] (Copyright 2017 Wiley)
- Figure 11 Top view of perovskite film on TiO2-Cl (a) [97] (Copyright 2017 Science); Schematic illustration of device structure ITO/TiOx-Au-NPs/perovskite/HTL/Ag (b) (Au-NPs are sandwiched between two TiOx layers [100], Copyright 2015 Wiley); Cross-sectional SEM image of c-TiO2/a-SnO2 ETL (c) [101] (Copyright 2018 Wiley)
- Figure 12 J-V curves of PSCs using SnO2 and SnO2/PCBM ETLs under reverse and forward voltage scanning (a) [111] (Copyright 2016 RSC); J-V curves of PSCs using EDTA, SnO2 and E-SnO2 ETLs with inset showing device configuration (b) [113] (Copyright 2018 Nature)
- Figure 13 Schematic illustration of energy levels of ZnO-ETLs and perovskite film (a) [121] (Copyright 2018 ACS); Schematics of perovskite films annealed on the top of ZnO ETL and MLG/ZnO ETL (b) [122] (Copyright 2019 RSC)
- Figure 14 Device structure of inverted planar heterojunction PSCs: ITO/PTAA/MAPbI3/PCBM/C60/ BCP/Al [172] (Copyright 2016 Nature)
J. Cent. South Univ. (2020) 27: 1104-1133
DOI: https://doi.org/10.1007/s11771-020-4353-7
Solution-processed perovskite solar cells
CHANG Jian-hui(常建辉), LIU Kun(刘坤), LIN Si-yuan(林思远), YUAN Yong-bo(袁永波),
ZHOU Cong-hua(周聪华), YANG Jun-liang(阳军亮)
Hunan Key Laboratory for Super-microstructure and Ultrafast Process, School of Physics and Electronics, Central South University, Changsha 410083, China
Central South University Press and Springer-Verlag GmbH Germany, part of Springer Nature 2020
Abstract:
Perovskite solar cells (PSCs) have emerged as one of the most promising candidates for photovoltaic applications. Low-cost, low-temperature solution processes including coating and printing techniques makes PSCs promising for the greatly potential commercialization due to the scalability and compatibility with large-scale, roll-to-roll manufacturing processes. In this review, we focus on the solution deposition of charge transport layers and perovskite absorption layer in both mesoporous and planar structural PSC devices. Furthermore, the most recent design strategies via solution deposition are presented as well, which have been explored to enlarge the active area, enhance the crystallization and passivate the defects, leading to the performance improvement of PSC devices.
Key words:
Cite this article as:
CHANG Jian-hui, LIU Kun, LIN Si-yuan, YUAN Yong-bo, ZHOU Cong-hua, YANG Jun-liang. Solution-processed perovskite solar cells [J]. Journal of Central South University, 2020, 27(4): 1104-1133.
DOI:https://dx.doi.org/https://doi.org/10.1007/s11771-020-4353-71 Introduction
Organic-inorganic hybrid halide perovskite materials have recently become one of the most intensively investigated optoelectronic materials due to their merits of high absorption coefficient [1], high carrier mobility [2, 3], long electron-hole diffusion length [4, 5], tunable direct bandgap [6, 7], and low exciton binding energy [8, 9]. The power conversion efficiency (PCE) of perovskite solar cells has been significantly jumped from 3.8% to 25.2% in less than 10 years [10-17]. Not only is this PCE nearing that of market leading single crystal silicon cells, but also the solution processability and compatibility with printing and roll-to-roll (R2R) manufacturing process offer the potential for low-cost photovoltaic technology.
In general, organic-inorganic hybrid halide perovskite materials possess an ABX3 structure, where A-site typically utilizes methylammonium (MA+),formamidine (FA+), or Cs+, B-site can be Pb2+ or Sn2+ and X-site is halide ion (Cl-, Br-, I-). There are several methods for depositing the perovskite light absorbing layer in PSC devices, such as solution process, co-evaporation, chemical vapor deposition (CVD), close space sublimation [18-21]. As compared with other types of deposition methods, the solution process exhibits the advantages of low cost and the compatibility with large-scale, high-throughput R2R manufacturing processes.
PSCs are typically classified into two types of device architectures, i.e., conventional structure (n-i-p) and inverted structure (p-i-n)(Figures 1(a)-(c)), and conventional structural PSCs include mesoporous structure and planar structure, of which organic small molecular or polymer materials are normally used as the hole transport layer (HTL). For the mesoporous structural PSCs (meso-PSCs), the electron transport layer (ETL) includes both the compact layer and the mesoporous layer. However, the fabrication of mesoporous metal oxides typically requires high sintering temperature over 400 °C to create the conductive phase, limiting the development of flexible meso-PSCs. While the planar structural PSCs do not require the induction of mesoporous frameworks and high-temperature sintering, which makes the fabrication of PSCs be more diverse at the temperature lower than 150 °C. The state-of- the-art PCEs of planar n-i-p PSCs are more than 23%, of which SnO2 is used as the dense ETL [12]. Due to the advantages of low-temperature solution processing, low hysteresis effects and compatibility with flexible substrates, the inverted planar PSCs have drawn tremendous attention and show high efficiency close to that of the meso-PSCs [22-24]. Many efforts in device fabrication, composition engineering, post-treatment engineering and material optimization have been devoted to optimizing the efficiency and properties of the inverted planar PSCs [25].
This review first provides an overview on solution-processed meso-PSCs (Section 2), including PSCs using mesoporous TiO2 (mp-TiO2) or doped mp-TiO2 as electron-transporting materials, as well as PSCs using mesoporous Al2O3 (mp-Al2O3) as the scaffold. Sections 3 describes solution- processed perovskite layer for planar structural PSCs, including spin coating deposition and scalable deposition such as doctor blading, slot-die coating, spray coating and other deposition techniques. Section 4 summarizes the ETL and HTL for solution-processed conventional planar PSCs, while Section 5 summarizes the HTL and ETL for solution-processed inverted planar PSCs. Section 6 summarizes the solution-processed top electrodes. Section 7 provides concluding remarks and summarizes the outlook.
2 Solution-processed meso-PSCs
2.1 PSCs using mp-TiO2 as electron-transporting material
Following the pioneered works in dye- sensitized solar cells, PSCs were successfully fabricated based on mp-TiO2 scaffold. Besides the first report by MIYASAKA in 2009 using perovskite nanocrystallites as “sensitizers” and also the liquid-state electrolyte as hole-transporting material [10], GRATZEL et al [26] deposited perovskite film directly on mp-TiO2 by sequential processes in 2013. The PbI2 solution was first deposited onto mp-TiO2, and subsequently transformed into the MAPbI3 perovskite by soaking it in MAI solution. As shown in Figure 2(a), perovskite film of one-step process has a large grain size, but the distribution is uneven, and the coverage is relatively low. However, perovskite film prepared from sequential two-step deposition has uniformly-distributed grain size, with higher coverage (Figure 2(b)). The device achieved the PCE of 15.0% with short-circuit current (Jsc) of 20.0 mA/cm2, open-circuit voltage (Voc) of 0.993 V, and fill factor (FF) of 0.73 (Figure 2(c)).
Figure 1 Schematic illustration of PSCs with conventional mesoporous structure (a), conventional planar structure (b), and inverted planar structure (c)
Figure 2 SEM images of perovskite films fabricated via one-step (a) and two-step (b) sequential deposition on FTO substrate, (c) J–V curves for a best-performing cell measured at a simulated AM 1.5G solar irradiation of 96.4 mW/cm2 (solid line) and in the dark (dashed line) [26] (Copyright 2013 Nature)
In 2016, GRATZEL et al [27] embedded small and oxidation-stable rubidium (Rb+) cation into “A site” and created a cationic blended perovskite. The results indicated that Rb+ could modify the crystal lattice of perovskite and favor the growth of perovskite black-phase, leading to the PCE up to 21.8% with Jsc of 22.8 mA/cm2, Voc of 1.18 V and FF of 0.81. In addition, excellent photo-stability was achieved, and 95% of its initial efficiency was retained though the RbCsMAFA device had been thermal treated at 85 °C for 500 h.
The PCE of mp-TiO2 based PSCs was further improved via the modification in HTL material. In 2018, SEO et al [15] synthesized a fluorene- terminated hole-transporting material (N2,N2,N7, N7`-tetrakis(9,9-dimethyl-9H-fluoren-2-yl)-N2,N2′, N7,N7′-tetrakis (4-methoxyphenyl)-9,9`-spirobi [fluorene]-2,2`,7,7`-tetraamine, which exhibited a better fine-tuned energy level and higher glass transition temperature, as compared with the typical HTL material 2,2’,7,7’-Tetrakis-(N,N-di-4- methoxyphenylamino)-9,9-spirobifluorene (Spiro- OMeTAD). Thus, the PCE up to 23.2% could be achieved with Jsc of 24.91 mA/cm2, Voc of 1.144 V and FF of 0.883. The devices also exhibited better thermal stability.
Although meso-PSCs with metal electrode have achieved optimistic PCEs, these devices suffer from the stability issues due to the corrosion behavior of perovskite materials. Thus, carbon- electrode was developed to be used as the electrode instead of metal ones. In 2014, HAN et al [28] filled the mesoscopic skeleton of “mp-TiO2/mp- ZrO2/C” with precursor containing MAI, HOOC(CH2)4NH3I (5-AVAI) and PbI2 in the solvent of γ-butyrolactone (Figure 3(a)). The 5-AVAI was found to play a key role in carbon-electrode PSCs, in which the group of “—COOH” in 5-AVAI could bind to the inner surface of the mesoporous skeleton and the terminal group of “—NH3+” could serve as “nucleation site” for the subsequent growth of perovskite crystallites. As a result, “anchoring effect” appears, and it can enhance the loading behavior of perovskite crystallites on the skeleton. The TEM images (Figures 3(c) and (d)) show that uniform and compact loading could be achieved after the addition of 5-AVAI, leading to the upgraded Jsc and PCE, as shown in Figure 3(b). Such strategy has been verified as well when using TiO2 nanoparticle-binding carbon-film as the top electrode in the hole-conductor-free meso-PSCs [29-32].
2.2 PSCs using doped mp-TiO2 as electron- transporting material
Hysteresis is one of the key issues in PSCs, especially for those based on mp-TiO2. The doping process is developed to treat mp-TiO2 for hopefully decreased hysteresis. GRATZEL et al [33] doped mp-TiO2 using Li and hysteresis behavior was greatly decreased (Figures 4(a) and (b)). X-ray photoelectron spectrometric (XPS) results showed that Li-treatment could induce the conversion of partial Ti4+ to Ti3+, as shown in Figures 4(c)-(f). Besides, the concentration of sub-bandgap states was reduced as well (Figure 4(g)), which improved the electron transport within mp-TiO2 (Figure 4(h)). Besides Li, other elements have also been used as the doped materials, such as Nb [34], Y [35], Co [36].
Figure 3 Schematic of fully printable carbon-electrode meso-PSCs (a) (The mesoporous layers of TiO2 and ZrO2 have a thickness of ~1 and 2 mm, respectively, and are deposited on a FTO-covered glass sheet. They are infiltrated with perovskite by drop-casting from solution); (b) J-V curves under simulated AM 1.5 solar irradiation at an intensity of 100 mW/cm2 measured at room temperature; (c) and (d) TEM images of MAPbI3 and (5-AVA)x(MA)1-xPbI3 [28] (Copyright 2016 Science)
Though TiO2 has been widely used in PSCs as a famous ETL, the active photocatalysis effect is thought as a shortage in application of optoelectronic devices, especially for solar cells. Such feature is mainly caused by the UV sensitivity of TiO2. In 2017, LIU et al [29] observed a kind of irreversible light-soaking behavior in mp-TiO2 based PSCs. With scanning round goes on, the PCEs increases, and the value returns to its starting point even though the device was recovered in dark for 1 to 4 days. Such behavior is different from the previously reported “reversible behavior” [37], it was caused by UV light-induced oxygen vacancies in TiO2. The oxygen vacancies lead to “N-type” doping effect and increase the film conductivity and improve interfacial charge transfer.
2.3 PSCs using mp-Al2O3 as scaffold
Besides mp-TiO2, other mesoporous materials have been used as the scaffold in PSCs as well. In 2012, SNAITH et al [38] reported a “meso- superstructured solar cell” using mp-Al2O3 instead of mp-TiO2 (Figure 5(a)). As the Al2O3 was applied in the device, the Voc was over 200 mV higher than the mp-TiO2 based PSCs (Figure 5(b)). For TiO2-based PSCs, the electrons transport to the TiO2 and the holes transfer to the Spiro-OMeTAD after light absorption in perovskite. For Al2O3-based PSCs, the electrons remain in perovskite phase until they are collected by compact TiO2-coated FTO electrode, and they must transport throughout the perovskite thickness. Therefore, the Al2O3 scaffold did not act as an n-type layer in PSCs. The charge generation in both kinds of PSCs was performed by photoinduced absorption (PIA), as shown in Figure 5(c). The PIA spectrum of TiO2-based perovskite revealed feature in near-IR due to the free electrons in Ti, but the Al2O3-based perovskite exhibited no PIA signal, confirming the role of “scaffold”. Furthermore, it was proved that the charge collection in Al2O3-based PSCs was faster by a factor of over 10 than that of TiO2-based PSCs, indicating that the electron diffusion was faster through the perovskite than the n-type TiO2 (Figure 5(d)).
Figure 4 Cross-sectional SEM image of PSCs (a); J-V curves of PSCs with (red) and without (black) Li doping (b); doped mp-TiO2 layer for the O 1s peaks (c) and the undoped control (d); Ti 3s and Li 1s peaks slightly visible at 55 eV for Li-doped (e) and the signal for the undoped TiO2, which reveals the absence of the peaks related to Ti 3s (dashed line at 61.5 eV) and Li 1s, dashed line at 54.9 eV (f); Charge transport lifetime as function of the Jsc prepared with (red) and without (black) Li-doped TiO2(g); Charge extracted at open circuit as function of the voltage for no treated samples (black) and Li-doped samples (red) [33] (h) (Copyright 2016 Nature)
Figure 5 Schematic illustrating charge transfer and charge transport in a perovskite-sensitized TiO2 solar cell (left) and a noninjecting Al2O3-based solar cell (right) (a); J-V characteristics for Al2O3-based cells (red solid trace crosses for high efficiency of Al2O3-based cells, red dashed line with crosses for high Voc of Al2O3-based cells, black trace with circles for a perovskite-sensitized TiO2 solar cell, purple trace with squares for a planar-junction diode with structure FTO/compact TiO2/MAPbI2Cl/Spiro-OMeTAD/Ag) (b); PIA spectra of mp-TiO2 films (black circles) and Al2O3 films (red crosses) coated with perovskite with (solid lines) and without (dashed lines) Spiro-OMeTAD hole transporter (c);Charge transport lifetime determined by small-perturbation transient photocurrent decay measurement of perovskite-sensitized TiO2 cells (black circles) and Al2O3 cells (red crosses), both with lines to aid the eye (Inset shows normalized photocurrent transient for Al2O3 cells (red trace with crosses) and TiO2 cells (black trace with circles)) (d) [38] (Copyright 2012 Science)
3 Solution-processed perovskite layer for planar PSCs
Perovskite is a kind of materials with chemical formula ABX3 (A=MA+, FA+, Cs+; B=Pb2+, Sn2+; X=I-, Br-, Cl-), has aroused worldwide attention as excellent photoactive materials due to the impressive intrinsic optoelectronic properties including high absorption coefficient, long charge recombination lifetime, facile deposition, large carrier diffusion length and tunable bandgap, etc [13, 39-41]. So far, remarkable progress has been made in enhancing the efficiencies and stabilities of the PSCs. The enhancement is largely attributed to the improved crystallization, morphology, surface coverage of perovskite films owing to innovations in deposition techniques or modified strategies of perovskite films [13, 40, 42-45]. Therefore, enormous deposition techniques and optimization strategies including modifications and post treatments have been widely studied.
3.1 Spin coating deposition
3.1.1 One-step deposition
One-step solution deposition is widely used to fabricate perovskite film, in which a γ-butyrolactone (GBL), dimethylformamide (DMF) or dimethyl sulfoxide (DMSO) solvent solution containing mixed MAI and PbI2 precursors is directly deposited onto substrates [25]. JENG et al [46] reported the first inverted PSCs based on the perovskite films prepared by spin-coating a DMF precursor solution of equimolar MAI and PbI2, achieving a 3.9% PCE. Through composition engineering, the perovskite MAPbI3-xClx prepared by spin-coating of MAI and PbCl2 (3:1 molar ratio) in a DMF solution was utilized as the photoactive layer of inverted PSCs, delivering the PCE up to 10% by SNAITH’s group [25] and up to 11.5% by YANG’s group [22], respectively.
Furthermore, additives engineering is proved to be another efficient strategy to improve film quality. For example, HEO et al [47] demonstrated a hysteresis-less inverted PSCs with a PCE of 18.1% by using hydroiodic acid (HI) as the additive, leading to pinhole-free dense MAPbI3 films. CORREA-BAENA et al [48] reported a PSCs- based Rb-containing mixed cationic perovskite, of which the hysteresis was suppressed and the carrier lifetime and Voc were increased. The addition of as little as 1% RbI in precursor solution could suppress the excess PbI2 in the film. A champion stabilized efficiency of 20.3% for SnO2 based planar PSCs was achieved.
Poor device performance based on one-step spin-coating deposition mainly arises from non- continuous rough perovskite films, which may be due to the fast-uncontrollable film crystallization. Leakage current derived from poor morphology of perovskite films results in large device efficiency loss and poor device reproducibility [49, 50].
3.1.2 Two-step deposition
To control grain size and crystallization of perovskite films more effectively, various two-step solution deposition techniques have been intensively studied. BURSCHKA et al [26] reported a sequential deposition process of perovskite films in meso-PSCs, in which PbI2 dissolved in a DMF solution is firstly introduced into the mesoporous metal oxide films and subsequently transformed into the perovskite after tens of seconds by dipping it into a solution of MAI in iso-propyl alcohol (IPA). YANG’s group [51] reported another two-step solution deposition, named vapor-assisted solution deposition, for fabricating highly efficient planar PSCs. The resulting perovskite films with full surface coverage, small surface roughness and large grain size were obtained via in-situ reaction of the as-deposited film of PbI2 with MAI vapor. The corresponding conventional planar PSCs with the structure configuration of FTO/TiO2/perovskite/ HTL/Ag achieved a PCE of 12.1%.
BEIN et al [52] prepared MAPbI3-xClx film by immersing the PbI2 film in a heated solution of a mixture of MAI and MACl in IPA. They highlighted the criticality of chloride in MA lead halide perovskite via a controlled MACl into the MAI immersion solution. The photoluminescence lifetime of the photoexcited species in the device reaches values exceeding 300 ns influenced by the presence of chloride. The resulting devices based on TiO2 ETL exhibited the PCE approaching 15%. However, the perovskite films fabricated by this immersion process consisted of discontinuous perovskite particles, leading to a low coverage film and deteriorate device performance.
Apart from fabricating the meso-PSCs and conventional planar PSCs, a modified two-step solution deposition, named interdiffusion method, was developed in the inverted planar PSCs (Figure 6). The deposition and thermal annealing process at a low temperature of less than 150 °C resulted in full interdiffusion of spun PbI2/MAI stacking bilayers [53]. Based on the interdiffusion, homogeneous, uniform and pin-hole free MAPbI3 films formed and an efficient inverted device with a PCE up to 15.4% was obtained. Later, BI et al [54] studied the influence of wettability of various HTL materials such as PEDOT:PSS, polyvinyl alcohol (PVA), crosslinked compact-OTPD on the perovskite film morphology of inverted devices.
Figure 6 Schematic of interdiffusion deposition (a); SEM images of PbI2 film (b) and annealed MAPbI3 film deposited by an interdiffusion deposition (c) and one-step deposition (d) [53] (Copyright 2014 RSC)
They found that non-wetting HTLs benefited the formation of perovskite films with larger grain sizes, which significantly reduced the trap density of perovskite films and boosted the PCE to 18.3%. In another study, by inserting a tunneling layer, such as insulating polystyrene (PS), Teflon, or polyvinylidene-trifluoroethylene copolymer (PVDF- TrFE), between perovskite and ETL, the interface carrier recombination was suppressed due to the hole blocking effect of the interfacial insulating polymer. By using this nonlattice-matching structure, the PSCs fabricated by two step spin-coating achieved a high PCE of 20.3% and exhibited water-resistant [55].
3.2 Scalable deposition
The past few years have witnessed the successful application of spin-coating deposition in the fabrication of highly efficient PSCs. However, spin coating is not suitable for scalable production due to non-uniform large-area films resulted from centrifugal force. Therefore, various scalable deposition techniques have been proposed to satisfy the potential mass production of PSCs without the sacrifices of device performance. Some representative scalable techniques including doctor- blade coating, slot-die coating, spray coating will be briefly discussed below [19, 56-58].
3.2.1 Doctor-blade coating
Doctor-blade coating is a simple, low-cost, high-throughput deposition process for the fabrication of planar PSCs. In order to deposit a uniform and pin-hole free thin film, a precursor solution of PbI2 and MAI dissolved in a specific solvent is dropped onto substrate, and subsequently swiped by a blade at a certain speed [57](Figure 7(a)). In this deposition, the film thickness can be controlled by the concentration of precursor solution, the gap between blade and substrate, the sweeping speed of blade across substrate, surface tension force, annealing temperature, etc [59-63].
JEN et al [56] firstly reported the doctor- bladed PSCs based on the uniform MAPbI3-xClx perovskite films. The doctor-bladed perovskite films were deposited in ambient condition, achieving a self-assembled large crystalline domains and improved device stability. Later, the impact of doctor-blading speed on the perovskite film morphologies was studied. For example, HUANG’s group [64] chose a large speed of over 50 mm/s in the Landau–Levich region and incorporated surfactants into the precursors solution, enabling doctor-blading of smooth perovskite films with small surface roughness. They found that a very small amount of surfactants dramatically altered the fluid drying dynamics, increased the adhesion of the perovskite ink to the underlying non-wetting substrates and passivated the charge traps. The resulted PSCs achieved PCEs over 20% in small area and module efficiencies of 15.3% measured at aperture area of 33.0 cm2. Similar to PSCs based on spin-coated perovskite films, PSCs based on doctor-bladed perovskite films exhibit improved performance in efficiency and stability through composition engineering [64-68]. For example, a bilateral alkylamine (BAA) additive was incorporated into the perovskite doctor-blading process and could passivate the grain surface effectively. This defect-passivation effect resulted in the enhancement of device performance and stability, achieving a PCE of 20% (aperture,1.1 cm2) and keeping 90% of the initial efficiency after 500 h under light.
3.2.2 Slot-die coating
Slot-die coating is a R2R compatible process for processing large-area PSCs due to its continuous ink supply. It involves the transportation of perovskite solution from the ink reservoir of slot-die head to the substrate and the formation of perovskite film under elevated temperature (Figure 7(b)) [69].
Based on the modification of a 3D printer, slot-die coating was successfully used in the scalable fabrication of PSCs after the point nozzle was substituted by a mini slot-die head with metal shims [58]. Later, a PC-controlled system with temperature-control enabled the fully slot-die coated PSCs with exception of evaporated metal electrode. Furthermore, combined with gas- quenched treatment, this process achieved more dense and uniform PbI2 films [70]. In addition, ZHU et al [71] demonstrated a slot-die coating process for the fabrication of PSCs at room temperature and in ambient environment, achieving a reverse J-V sweep PCE of 18%. In particularly, a robust perovskite precursor ink with long wet-film processing wind used in this slot-die coating process could be also utilized in other deposition methods such as doctor-blade coating, spray- coating and spin coating.
3.2.3 Spray coating
As another scalable deposition approach, spray coating exhibits great potential in the fabrication of thin films on varied substrates. Spray coating process involves the solution atomization into droplets under ultrasound, droplet spray under gas flow and material crystallization with solvent evaporation (Figure 8(a)) [62, 72].
Figure 7 Schematic illustration of doctor-blade coating (a) and slot-die coating (b) [18] (Copyright 2019 Wiley)
Figure 8 Schematics of spray coating (a) [51] and micro-gravure printing (b) [74] (Copyright 2019 RSC)
BARROWS et al [53] introduced one-step spray coating of the blend precursor solution containing MAI and PbCl2 into the MAPbI3-xClx film deposition. Followed by thermal annealing, the PSCs achieved a PCE of 11%. Furthermore, by adjusting the composition of DMF and GBL mixed solvents, the largest MAPbI3-xClx crystal grains at a 8:2 volume ratio of DMF:GBL were obtained via spray coating due to the flux balance, resulting in a high PCE up to 18.3% in inverted planar PSCs [72]. However, spray coating may be impractical for the potential use due to material loss and low-resolution patterning.
3.2.4 Other deposition techniques
On the pathway toward mass production of large-area PSCs and modules, gravure printing combined with scalable R2R fabrication might be a possibility (Figure 8(b)). The advance of well- defined edges, controllable thickness and high material utilization make the gravure-printing are the most compatible techniques with R2R process in large-scale production. Gravure printing is frequently used for the deposition of patterned structures in polymer solar cell modules [73, 74], and the potential of gravure printing as a scalable printing to fabricate perovskite devices has been demonstrated. HU et al [61] reported high oriented, large-area, ultra-long MAPbI3 perovskite nanowires fabricated by a large-scale R2R micro-gravure printing process on flexible polyethylene terephthalate (PET) substrate in ambient environment. Thereafter, TONG et al [75] reported high-quality Cs-doped triple cation perovskite film fabricated by R2R micro-gravure printing onto PET substrate. Furthermore, GONG et al [76] fabricated a SnO2 ETL on a PEN-based ITO substrate used R2R micro-gravure printing process and deposited triple-cation (MA/FA/Cs) perovskite film used a R2R sequential deposition process, i.e., first Cs-doped PbI2 was deposited by R2R micro- gravure printing and then triple-cation halide films was deposited by R2R slot-die coating. The PCEs of triple-cation flexible PSCs fabricated using R2R sequential printing was up to 10.56%.
In addition, various other attempts, such as inkjet printing [18, 77, 78], screen printing [79, 80], hot-casting [81], soft-cover deposition [82, 83], have also been used to fabricate PSCs.
3.3 Post-treatment
The perovskite film morphology involving surface coverage, grain size, crystallinity and uniformity, etc., is crucial to the device performance. Post-treatment, as an efficient strategy of enhancing film quality, have been intensively studied [19]. The schematics of some representative post-treatments including solvent annealing, Ostwald ripening and methylamine gas treatment are illustrated in Figure 9.
The solvent-annealing treatment is an effective strategy for enhancing the crystallinity and quality of MAPbI3 films [84, 85]. SEOK’s group designed a novel solvent-engineering strategy, through which the use of a mixed solvent of GBL and DMSO followed by a toluene drip, extremely homogeneous perovskite layer via a MAI-PbI2-DMSO intermediate phase could be formed. The PSCs using this solvent-engineering technology reached a certified PCE of 16.2% [86]. HUANG’s group utilized another solvent annealing treatment of dissolving the PbI2 and MAI at the interfaces by DMF vapor to increase the MAPbI3 grain size and crystallinity, leading to performance enhancement with the PCE over 14% and the diffusion length over 1 μm resulting from deeper diffusion of precursor ions and molecules than in all-solid state [84]. A cryogenic process of cooling as-cast precursor films down and subsequently blow-drying with nitrogen gas could control the perovskite film deposition, achieving anti-solvent-free highly efficient PSCs [87].
Figure 9 Schematics illustrating representative post-growth treatments for perovskite films, i.e., Ostwald ripening, solvent annealing and methylamine gas treatment [19] (Copyright 2018 Nature)
Interestingly, the MAPbI3 shows a unique soft-matter nature. A surface tuning strategy of modifying the interface between perovskite and HTL by surface agents employed in the fabrication of perovskite films without inducing the formation of recombination or degradation centers [88]. A methylamine (CH3NH2) induced defect-healing (MIDH) behavior, which resulted from the reversible reaction of CH3NH3PbI3 and CH3NH2 and the formation of an intermediate CH3NH3PbI3·xCH3NH2 liquid phase, was observed. The performance of PSCs treated by MIDH exhibited a dramatically enhanced efficiency due to film morphology improvement [89]. On the other hand, ZHAO et al [90] reported an Ostwald ripening process for the formation of compact, large-grain and pinhole-free MAPbI3-xIx films. The MABr treatment via spin coating with proper concentration was proved to be an effective approach to construct high-quality perovskite films with larger grain size.
As mentioned above, uniform and pin-hole free perovskite films with large grain size are crucial to the formation of high-performance PSCs for the reduced defects caused by grain boundaries and density of trap states. Therefore, defect passivation can effectively improve the film quality, thereby obtaining the highly efficient PSCs. The (6,6)-phenyl C61-butyric acid methyl ester (PCBM) layer spun onto the light absorbing layer can passivate the charge trap states on the surfaces and boundaries of perovskite materials, yielding a hysteresis-free device with minimized charge recombination and enhanced efficiency [91,92]. As compared to PCBM passivation strategy, HUANG et al [93] reported one more effective ionic defect passivation strategy by utilizing quaternary ammonium halides (QAHs) in the fabrication of perovskite film, leading to remarkably increased Voc and improved efficiency due to reduced surface trap density and long carrier lifetime. The PCEs of PSC devices containing MAPbI3 and FA0.85MA0.15Pb(I0.85Br0.15)3 were 20% and 21%, respectively.
In addition, an exciting surface defect passivation strategy was reported by YOU’s group, and the certificated PCE of planar PSCs increased up to 23.32% with a high Voc of 1.18 V. They developed an organic halide salt, phenethylammonium iodide (PEAI), by which the defects of FA/MA mixed perovskite films were effectively passivated and non-radiative recombination caused by defects became much less [12]. The above post-treatment is a popular strategy to increase Voc. LIN et al [94] reported a n-butylamine (BA) treatment to construct a 2D/3D stacking structures for the fabrication of highly stable PSCs. It is different to n-butylammonium iodide (BAI) treatment, (BA)2PbI4 produced by reaction of BA with MAPbI3 has better protection due to its more organic ligands and the mixture of 2D perovskites, leading to the PCE to 19.56%.
4 ETL and HTL for solution-processed conventional planar PSCs
4.1 ETL for solution-processed conventional planar PSCs
The ETL materials usually play an essential role in the performance of PSCs. Qualified ETL materials must meet the following conditions: (1) good energy-level alignment with perovskite for efficient charge transfer and hole blocking; (2) high electron mobility to guarantee fast electron transport within the ETL; (3) high transmittance to ensure full use of sunlight; (4) stability; (5) low cost and easy processing [39, 95-97].
4.1.1 TiO2 planar ETL
Generally, TiO2 was used as ETL in planar PSCs. In 2014, EPERON et al [40] first reported efficiencies above 10% in fully solution-processed MAPbI3-xClx PSCs with planar heterojunction architecture and then reported the PCEs up to 14.2% in FA lead trihalide PSCs [41]. Initially, the low PCE of planar n-i-p PSCs was due to the poor morphology of perovskite film because of the lack of a mesoporous layer and the high defect density at the perovskite/TiO2 interface, which lead to the short diffusion length and carrier lifetime, as well as large non-radiative recombination loss at the interface.
Therefore, a lot of work was focused on the modification of perovskite morphology and passivated perovskite/TiO2 interface defects. Additive engineering is a common strategy to improve the morphology of perovskite films [42, 43]. ZHOU et al [44] presented a possible microscopic mechanism concerning the perovskite nucleation and grain growth in the presence of weak coordination additives through introducing the weak coordination molecule acetonitrile (ACN) as the additive into the PbI2/DMF solution. The perovskite film displayed gradually enlarged grain size by increasing its concentration, with the grain size in the range of a few hundreds of nanometers to over 1 μm (Figure 10(a)). Finally, the planner configuration PSCs of ITO/TiO2/Perovskite/Spiro- OMeTAD/Au with ACN-aided perovskite films achieved an average PCE of 17.43% and the best PCE of 19.7%.
CHEN et al [45] proposed a novel approach to modulate the nucleation and growth of perovskite crystals in planar PSCs by intermixing precursor- capped inorganic nanoparticles of PbS (Figure 10(b)). Through this intermixing-seeding growth technique, substantial morphological improvements were realized in perovskite films, such as increased crystal domains, enhanced coverage, and uniformity. ZHOU et al [98] reported a MA/EtOH (CH3NH2/ethanol) additive-based solution process to fabricate high-quality perovskite films. These led to substantial reduction in defect density and extended charge carrier diffusion lengths in the resulted film (Figure 10(c)). Subsequently, the planar PSCs were fabricated by thick perovskite absorber (~650 nm) achieved a PCE of 19.01%.
However, it was found that the TiO2/perovskite owns the charge barrier. Thus, it would lead to charge accumulation and inefficient charge transfer at the interface [39]. In addition, the TiO2 film normally contains many oxygen vacancies at the interface that could reduce the long-term stability of solar cell devices. In order to overcome the limitations of TiO2, a few strategies have been implemented. TAN et al [99] reported a contact-passivation strategy using chlorine-capped TiO2 (TiO2-Cl) colloidal nanocrystal film that mitigates the interfacial recombination and improves interface binding in low-temperature planar PSCs. The smooth, pinhole-free mixed cation-halide perovskite FA0.85MA0.15Pb2.55Br0.45 films with uniform and large grains were formed on TiO2-Cl (Figure 11(a)), and the PSCs reached a PCE of 21.4%. SUN et al [100] developed a sandwiched TiOx-Au-TiOx composites structure to act as the electron conductor to improve the efficiency (Figure 11(b)). Au-NPs can enhance the conductivity and decreased surface potential of TiOx film. KONG et al [101] reported a planar structure PSCs based double layer structural ETL, i.e., TiO2 compact layer and SnO2 amorphous layer, achieved a maximum PCE of 21.4% (Figure 11(c)). After modification with SnO2, the PSCs based TiO2/ SnO2 ETL exhibited a good stability under the UV light because the SnO2 layer can shield the charge transfer passes.
4.1.2 SnO2 planar ETL
In order to further improve the PCEs of PSCs, SnO2 is used to replace TiO2 as ETL due to its deep conduction band and good energy level, high bulk electron mobility (up to 10-3 cm2 V-1 s-1), low- temperature process and excellent chemical stability [39, 102-104]. In recent years, most planar heterojunction PSCs used SnO2 as the ETL have achieved highly efficient PCEs [105-109]. In 2016, YOU et al [110] developed a regular n-i-p planar structural PSCs with solution-processed SnO2 nanoparticles as the ETL on ITO substrate, which was baked on a hot plate in ambient air at 150 oC for 30 min. The PSCs with a planar structure of ITO/SnO2/(FAPbI3)0.97(MAPbBr3)0.03/Spiro-OMeTAD/Au achieved a PCE close to 20%. Now, PSCs based SnO2 ETL have achieved the PCE as high as 23.3% [12].
Modifying SnO2 by adding dopants or additives can effectively increase the electron mobility of SnO2 and engineer energy levels that would better match with those of perovskites. YAN et al [111] developed a SnO2/PCBM ETL in planar PSCs. In the process of spin-coating perovskite precursor solution, the PCBM could be re-dissolved by DMF and DMSO, which would allow only ultra-thin PCBM to be retained at the interface and some dissolved PCBM infiltrate into perovskite grain boundaries. The PCBM in PSCs plays a role of passivate both the SnO2/perovskite interface and perovskite grain boundaries and promote electron transfer. The PSCs with an unpassivated SnO2 ETL achieved a PCE of 16.3% and with a SnO2/PCBM ETL achieved a PCE of 18.7% (Figure 12(a)). Fullerene C60 was also introduced onto SnO2 by CAROUS et al to partially fill the surface trap states in SnO2 and passivated both of the C60/perovskite and SnO2/C60 interfaces [112]. Meanwhile, the better energy alignment could effective suppression of charge recombination and thus enhanced Voc.
Figure 10 Top-view SEM images of perovskite films obtained by two-step spin coating using different ratios of ACN in DMF (a) [44] (Copyright 2016 Wiley); Schematic of the ligand exchange process and the proposed nucleation and growth routes of perovskite crystal films (b) (The assisted formation of lead-halide octahedrons in the vicinity of an MAI-capped PbS nanoparticle [45], Copyright 2016 RSC); Current density-voltage characteristics of devices with ITO/perovskite/Au configuration utilized for estimating the defect density in perovskite films (c) [98] (Copyright 2017 Wiley)
Figure 11 Top view of perovskite film on TiO2-Cl (a) [97] (Copyright 2017 Science); Schematic illustration of device structure ITO/TiOx-Au-NPs/perovskite/HTL/Ag (b) (Au-NPs are sandwiched between two TiOx layers [100], Copyright 2015 Wiley); Cross-sectional SEM image of c-TiO2/a-SnO2 ETL (c) [101] (Copyright 2018 Wiley)
LIU et al [113] realized an EDTA-complexed SnO2 (E-SnO2. EDTA: Ethylene diamine tetraacetic acid) ETL by complexing EDTA with SnO2 in planar PSCs (Figure 12(b)). As compared to normal SnO2, the electron mobility of E-SnO2 ETL increases by about three times. The PSCs with E-SnO2 ETL achieved PCE as high as 21.60%. HU et al [114] applied the commercially accessible SnO2 and home-made TiO2 NPs as a combined electron transport bilayer to achieve planar PSCs that acquired a high PCE of 20.50%.
Recently, new progress has been made in the modification of SnO2. HUI et al [115] used carboxylic-acid- and hydroxyl-rich red-carbon quantum dots (RCQs) to dope low-temperature solution-processed SnO2, which increased its electron mobility by ~ 20 times from 9.32×10-4 to 1.73×10-2 cm2/(Vs), and a champion PCE for n-i-p heterojunction PSCs up to 22.77% has been achieved. In addition, KCl [116] and graphitic carbon nitride (g-C3N4) quantum dots [117] could effectively passivate the defect at the ETL/ perovskite interface and eliminate the oxygen- vacancy-reduced trap centers. As the result, the PCEs of planar PSCs can exceed 22%.
4.1.3. ZnO planar ETL
ZnO is a promising ETL material due to its charge carrier mobility, carrier lifetime and electron diffusion coefficient that is orders of magnitude higher than that of TiO2 [118]. In 2016, HEO et al [119] fabricated low-temperature processable ZnO ETL for MAPbI3 PSCs with an average PCE of 17.6%. However, the chemical instability at the interface is a major challenge. The extended heat-treatment made the perovskite change from dark brown to right yellow due to ZnO induced proton-transfer reactions at the ZnO/perovskite interface, leading to eventual decomposition of the perovskite film into PbI2 [120]. Therefore, a range of approaches including optimizing deposition techniques, heteroatom doping and interface modification have been successfully established to enhance the stability of the ZnO/perovskite interface and the photovoltaic performance. AZMI et al [121] incorporated the alkali cations K+ into ZnO, which could improve the electron mobility, raise the Fermi energy level and passivate the defects of ZnO ETLs (Figure 13(a)). Thus, PSCs fabricated with K:ZnO ETL achieved the highest PCE of 19.9% and better stability. TAVAKOLI et al [122] demonstrated improved photovoltaic performance and stability by introducing monolayer graphene (MLG) at the interface between the ZnO ETL and perovskite absorber, resulting in stable PSCs with the PCE up to 19.81% (Figure 13(b)).
4.1.4 Other planar ETLs
There are some other binary ETL materials in addition to TiO2 and SnO2, including metal binary oxide, metal sulfide and selenide and metal ternary oxide. These materials exhibit some drawbacks regarding their application as the ETLs, but intriguing properties have been shown when integrated in PSCs.
Figure 12 J-V curves of PSCs using SnO2 and SnO2/PCBM ETLs under reverse and forward voltage scanning (a) [111] (Copyright 2016 RSC); J-V curves of PSCs using EDTA, SnO2 and E-SnO2 ETLs with inset showing device configuration (b) [113] (Copyright 2018 Nature)
Figure 13 Schematic illustration of energy levels of ZnO-ETLs and perovskite film (a) [121] (Copyright 2018 ACS); Schematics of perovskite films annealed on the top of ZnO ETL and MLG/ZnO ETL (b) [122] (Copyright 2019 RSC)
In2O3 can be used as an ETL material because it is an n-type semiconductor that possesses a wide band gap (~3.75 eV), a high electron mobility (~20 cm2 V-1 s-1) and good thermal stability. In 2016, QIN et al [123] fabricated In2O3/PCBM- based planar PSCs with a PCE of 14.8% via a sol-gel route to deposit In2O3 and modify the surface with the PCBM. By further optimizing the morphology of In2O3 ETL and offset the negative effect from pinholes, PSCs based In2O3 ETL have achieved the PCE higher than 16% [124, 125]. Nb2O5 has comparable electronic properties and chemical stability with TiO2. LIN et al [126] first reported Nb2O5 as the ETL in planar PSCs and achieved a PCE of 17.2%, exhibiting the great potential in PSCs. Furthermore, WANG et al [127] reported a low-temperature solution-processed Nb2O5 nanoparticle-formed film as the ETL in PSCs, and the highest PCE up to 20.22% was achieved with a very high Voc of 1.19 V. In addition, metal ternary oxide, such as Zn2SnO4 [127, 128], BaSnO3 [129, 130], SrSnO3 [131] were also employed as the ETLs in planar PSCs.
In addition to oxides, many metallic sulfides and selenides including CdS [132, 133], In2S3 [134], TiS2 [135, 136] and SnS2 [137] were also employed as ETLs in planar PSCs. DONG et al [132] used CdS thin films in planar PSCs and achieved a PCE of 16.5%. XU et al [134] successfully prepared In2S3 ETL via a solvent-thermal method and reached an impressive PCE of 18.83%. In 2018, 2D-TiS2 nanosheets were prepared by a simple solution exfoliation by YAN et al [135]. Using TiS2 thin films prepared from solution with an optimized concentration, PSCs show a high PCE of 17.37%. 2D-SnS2 is also a promising ETL in planar PSCs. Therefore, ZHAO et al [137] fabricated 2D SnS2-based PSCs and realized a PCE of 20.12%.
4.2 HTL for solution-processed conventional planar PSCs
The HTL is used to extract and transport the holes produced by perovskite and block the electron transport from perovskite to anode, as well as avoid carrier recombination. The HTL plays a critical role in boosting the PCEs of PSCs, and the commonly used HTLs in conventional PSCs, both mesoporous and planar structures, are Spiro-OMeTAD and poly[bis(4-phenyl)(2,5,6-trimethylphenyl)amine(PTAA). Spiro-OMeTAD is very commonly applied as the HTL in high-efficiency conventional structure PSC devices because of their suitable energy level, high solubility, amorphous character and high glass transition temperature. It has also been found to be the most suitable HTL for the fabrication of PSCs due to its facile nature and high device performance. In 2012, all-solid-state DSSCs based on MAPbI3 demonstrated a PCE of 9.7%, in which Spiro-OMeTAD was used as the HTM for the first time [138]. However, pure Spiro-OMeTAD exhibits lower conductivity and instability, several approaches are reported to overcome these issues. Bis(trifluoromethane)sul-fonimide lithium salt (LiTFSI) and tert-butyl pyridine (TBP) are widely doped in Spiro-OMeTAD. JIANG et al [12] reported the high PCE of 23.32% in PSCs with Spiro-OMeTAD HTL. Up to now, Spiro-OMeTAD is the most efficient HTL for PSCs, the only concern is the stability [106, 110, 139].
PTAA was reported to be a very effective HTL for high-performance PSCs. As a replacement of Spiro-OMeTAD, PTAA was first employed as the HTL in the conventional structural PSCs by SEOL et al and delivered a PCE of 12.0% [140]. SNAITH et al [141] achieved a best PCE of 23.6% under 14 suns illumination and retain its PCE more than 90% after 150 h under 10 suns with PTAA as the HTL. This has been proved to be an ideal candidate to tackle the instability of device, especially under the illumination and the elevated the temperature. However, in most cases, PTAA is used as the HTL in inverted structure PSC, which will be discussed later.
5 HTL and ETL for solution-processed inverted planar PSCs
The performance of perovskite films largely depends on the surface properties of HTL and ETL. Especially, whether the contact of the perovskite layer and HTL or ETL is good determines the extraction efficiency of the photogenerated charges, thereby greatly affecting the PCEs of planar PSCs. On the other hand, the matched energy levels between the perovskite layer and HTL or ETL are crucial to minimize the energy loss during charge extraction.
5.1 HTL for solution-processed inverted planar PSCs
5.1.1 Organic HTLs
As a highly conductive organic polymer blend with high work function (WF) and high optical transmittance [142-146], poly(3,4- ethylenedioxythio phene): poly(styrenesulfonate) (PEDOT: PSS) is the primary candidate that used as the HTL in planar PSCs due to its orthogonal solubility with perovskite precursor solutions [46, 147-154]. Although a reported high PCE above 18% for inverted PSCs with PEDOT:PSS as the HTL and MAPbI3 as the photoactive layer [47], in most of the cases, the PCEs of inverted PSCs are in a range of 14%-16% because of the energy loss at the PEDOT:PSS/MAPbI3 interface [53, 155-160]. This energy loss results from the mismatch between the Fermi-level of PEDOT:PSS (-5.2 eV to -4.9 eV) and the valence band (VB) of MAPbI3 (-5.4 eV) [161]. On the other hand, the absence of high lying lowest unoccupied molecular orbital (LUMO) level in PEDOT:PSS makes it fail to block electrons falling into the HTL. This feature of PEDOT:PSS results in obvious interface recombination and reduction of quasi-Fermi energy level splitting, and then produces a low Voc, which is another pathway for the energy loss [161]. Moreover, the acidic and hygroscopic PEDOT:PSS may induce instability caused by reactions with adjacent components and moisture filtration.
Considerable efforts have been devoted to the modification of PEDOT:PSS, such as film doping. For example, a polymer electrolyte PSS-Na or dopamine was doped into PEDOT:PSS to tune the WF of PEDOT:PSS, giving rise to highly efficient PSCs with a PCE above 15% [147, 162]. An inverted PSC with 2,3,5,6-Tetrafluoro-7,7,8,8- tetracyanoquinodimethane (F4-TCNQ) doped PEDOT:PSS as the HTL achieved an improved PCE from 13% to 17%, which resulted from the reduced HOMO level and enhanced electrical conductivity [163]. In addition, an interfacial engineering strategy by doping PEDOT:PSS with CsI was developed to enhance hole extraction from the perovskite layer to the HTL and suppress charge recombination at the perovskite/CsI-PEDOT:PSS interface, realizing inverted PSCs with excellent PCE of 20.22% [164]. Except doping strategy, the post-treatment of PEDOT:PSS with solution washing is another effective strategy to improve its conductivity and morphology by removing insulating PSS from PEDOT:PSS films. For example, OUYANG et al [165] reported that the PEDOT:PSS film treated by the MAI solution exhibited significantly enhanced conductivity.
In order to minimize the energy loss during the hole extracting from the perovskite layer to the HTL, the HTL materials with deep lying HOMO levels are desired to replace PEDOT:PSS as the HTL. Poly (N,N′-bis(4-butylphenyl)-N,N′- bis(phenyl)benzidine) (poly-TPD) with HOMO level of -5.2 eV was then selected as HTL material to extract holes because it can block electrons efficiently and can suffer from the lateral spin coating process of perovskite layer. The average efficiency of solution-processed inverted PSCs based on a perovskite layer sandwiched between a poly-TPD layer and a PCBM layer reached 13.8% [144]. The issue is that the HOMO of poly-TPD still does not match well with the VB of MAPbI3 [166], which leads to relatively low Voc value of 1.04 V [167].
As another popular hole transport materials in organic electronics [168, 169], PTAA has a deeper HOMO level of -5.25 eV than that of poly-TPD, making it more promising in inverted planar PSCs (Figure 14) [54, 170]. However, the poor hole mobility of PTAA (10-3-10-2 cm2/V·s) make it inefficient in hole extracting. The doping PTAA with F4-TCNQ was then investigated to reduce resistivity of HTL. Based on F4-TCNQ doped PTAA, inverted PSCs with thick PTAA exhibited enhanced Voc and FF without sacrifice of Jsc, boosting the PCE up to 17% [54, 171].
Figure 14 Device structure of inverted planar heterojunction PSCs: ITO/PTAA/MAPbI3/PCBM/C60/ BCP/Al [172] (Copyright 2016 Nature)
5.1.2 Inorganic HTLs
As alternatives to organic materials such as PEDOT:PSS and PTAA, inorganic HTL materials have drawn broad attention due to their better stability. P-type metal oxides, such as NiOx, Cu-doped NiOx, cuprous oxide (Cu2O) and copper oxide (CuO), etc., are frequently employed as the HTL materials since they have good electron blocking capability [173, 174].
As compared to PSCs based on PEDOT:PSS, inverted PSCs with NiOx as the HTL exhibited a remarkable enhanced photovoltaic parameters including Voc of 0.92 V, Jsc of 12.43 mA/cm2, FF of 0.68 and PCE of 7.8%, superior to that of PEDOT:PSS based PSCs with Voc of 0.62 V, Jsc of 9.39 mA/cm2, FF of 0.66 and PCE of 3.9% [175]. Similar to PTAA, NiOx also faced the issue of high resistivity due to its insufficient hole mobility. Adjusting its WF or doping NiOx by metal elements may be an effective approach to improve the PSCs performance. For example, a co-doping strategy of employing NixMgyNi1-x-yO as HTL material to extract the charge carriers was introduced, achieving a high PCE of 18.4% [176]. A PCE exceeding 20% could be achieved in inverted planar PSCs based on the high-quality HTL material of copper doped NiO (Cu:NiO). The PSCs with doping NiOx with 2,2′-(perfluoronaphthalene- 2,6-diylidene) dimalononitrile (F6TCNNQ) molecules as the HTL could achieved the PCE as high as 20% [177].
Due to the low-lying VBs of Cu2O and CuO that match well with the VB of the perovskite layer, a relative low energy loss existed in inverted PSCs with metal oxide based HTLs. DING’s group [174] reported the fabrication procedure of CuxO based inverted planar PSCs. Cu2O and CuO films were firstly prepared by a facile low-temperature deposition and used as HTLs, exhibiting remarkably enhanced Voc, Jsc and PCE. Apart from metal oxide, some materials such as graphene oxide (GO) [178], copper thiocyanate (CuSCN) [179], poly[(9,9-dioctyl-fluorenyl-2,7-diyl)-co-(4,4’-(N-(4-secbutyl-phenyl) diphenylamine)] (TFB) [180], etc., are also employed as the HTL materials in inverted PSCs. For instance, an ultrathin TFB film was employed as the HTL, achieving a PCE as high as 20% in inverted PSCs based on one-step coated perovskite layer.
5.2 ETL for solution-processed inverted planar PSCs
5.2.1 Fullerene and fullerene derivatives as ETL
In inverted PSCs, fullerene and its derivatives, such as ICBA, ICTA, and PC71BM, etc. [49, 85, 181], are the most popular ETL materials due to their excellent electron mobility and hole-blocking capability. Among them, PCBM is one representative electron acceptor [25, 166, 182, 183]. Firstly, fullerene-based materials were used in inverted PSCs to form a donor-acceptor (D-A) structure based on MAPbI3/C60 (or PCBM, etc.) planar heterojunction, the corresponding device achieved a maximum PCE of 3.9% for effective charge separation at the D-A interface [46]. With the rapid development of PSCs in terms of materials, structures and modification technologies, the PCEs of fullerene-based inverted devices have been continuously improved [53, 54, 84, 181].
The use of doped fullerene or fullerene derivatives as the ETL materials is an effective strategy to improve electron transport capability or improve film coverage. For example, oleamide [184], graphdiyne (GD) [185] and PS [55] was added into PCBM to realize electron transport enhancement. A charge-carrier-balance strategy that used the PCBM modified by poly(methylmethacrylate) (PMMA) additive and solvent-treated PEDOT:PSS as the ETL material and HTL material, respectively, was developed in inverted PSCs, achieving an enhanced PCE of 18.72% [186].
5.2.2 Non-fullerene ETLs
Although fullerene or fullerene derivatives are the dominant ETL materials in inverted PSCs, the poor film morphological stability and energy level adjustment restrict their broader applications. Therefore, an urgent need to develop new non-fullerene materials as the ETLs has promoted the emergency of new techniques or strategies.
Han’s group developed n-doped (n+) TiOx to extract selectively electrons, achieving highly efficient devices based on MAPbI3-PCBM architecture [176]. Perylenetetracarboxylic dianhydride (PTCDA), a typical perylene derivative, was employed as the ETL in inverted PSCs, achieving a maximum PCE of 16.5%. By inserting pyrene-substituted silicon phthalocyanine (SiPc- Py-2) into the perovskite/PTCDA interface, the PSCs boosted the PCE up to 19.2%, which resulted from the passivation effect [187]. A donor-acceptor (D-A) structured non-fullerene ETL materials, in which triphenylamine (TPA) and (3-cyano-4,5,5- trimethyl-2(5H)-furanylidene)malononitrile (3CN) were employed as the donor group and acceptor group, respectively, was utilized in the fabrication of inverted PSCs, exhibiting a comparable PCE of 19.2% with that of PCBM based PSCs [188].
6 Solution-processed top electrode
In PSCs, the top electrode can be prepared by a solution process. More importantly, PSCs with solution-processed top electrode can be semi- transparent, making them applicable tandem solar cells [189]. In addition, it also shows the feasibility for the low-cost, large-scale and high-throughput roll-to-roll production of solid-state devices. Up to now, various solution-processed top electrodes have been reported in PSCs, mainly metal-nanowire [190] and carbon materials [191].
It is strongly believed that the integration of Ag nanowires (AgNWs) network is the most promising solution processable electrodes for using in PSCs. 2016, DAI et al [192] fabricated PSCs using solution-processed AgNWs as transparent top electrode with markedly enhanced device performance, as well as stability, by evaporating an ultrathin transparent Au layer beneath the spin- coated AgNWs forming a composite transparent metallic electrode. However, when the AgNWs network is prepared by spin coating, the solvent in the AgNWs dispersion will corrode the perovskite layer, resulting in a decrease in the PCE and poor long-term stability. In addition, spin coating is not suitable for large-scale preparation. In order to reduce solvent damage to the perovskite films, spray coating was utilized onto the PSC devices. LEE et al [193] deposited AgNWs electrodes on the top of the Spiro-OMeTAD HTL by spray coating at moderate temperatures and achieved a PCE of 7.45%. ZHANG et al [194] reported a planar heterojunction PSC employing AgNWs as the top electrode and ZnO nanoparticles as the ETL. The PSC exhibited the highest PCE of 9.21%. It is worth nothing that the spray coated AgNWs are found to be weakly connected, which leads to the poor conductivity as well as low stability. To solve this problem, HAN et al [195] spray coated an additional layer of ZnO nanoparticle to form an AgNWs/ZnO composite electrode. The conductivity of the AgNWs/ZnO composite electrode was enhanced due to the improvement of the interconnection between AgNWs by filling the voids between AgNWs with ZnO nanoparticles. Finally, the high PCE of 13.27% with an averaged transmittance of 16.3% was achieved. In addition, inkjet printing can also be used to prepare AgNWs electrode. XIE et al [196] reported the preparation of transparent AgNWs top electrode for PSCs using inkjet printing process. By inserting a thin layer of polyethylenimine (PEI), the charge injection barrier between PC61BM and AgNWs electrode was minimized.
Carbon based materials have received much attention for use as conducting electrode in PSCs, due to their low cost and high stability. Importantly, it can be prepared by the solution processed. In addition, the carbon electrode is compatible with processes such as blade coating, slot–die coating, and screen printing. Thus, fully printing can be realized to prepare large-area PSCs [197]. MEI et al [28] printed carbon black/graphite composite material as the top electrode and achieved highly efficient and long-term stable conventional mesoporous structure PSCs [28]. Today, the PCE of such PSCs has reached 16.26% [198]. However, mesoporous carbon electrodes prepared by printing require high temperature annealing and are not compatible with flexible devices. Embedment carbon electrode is produced by a considerably simplified fabrication process which avoids high temperature, as a promising alternative to mesoporous carbon electrode. WEI et al [199] reported a new type of inkjet-printed carbon electrode. By designing the carbon with CH3NH3I ink to transform PbI2 in situ to CH3NH3PbI3, an interpenetrating seamless interface between the CH3NH3PbI3 active layer and the carbon hole- extraction electrode was instantly constructed, with a markedly reduce charge recombination as compared to that with the carbon ink alone. As a result, a considerably high PCE up to 11.60% was achieved. In addition, the process of covering the carbon electrode directly on the perovskite layer has attracted more and more attention, because this device structure and fabrication strategy is the simplest [200-202]. JIN et al [203] prepared a graphite/carbon black composite electrode by spin coating and fabricated PSCs with a structure of ITO/ZnO/CH3NH3PbI3/graphite/cabon black and the best device yielded 10.2% PCE.
7 Conclusions and outlook
In conclusion, the performance of PSCs fabricated by solution process has improved dramatically. By optimizing the composition of perovskite materials, selecting the appropriate charge transport materials and reasonably modifying the interface, the PCE of small-area PSCs have increased to the level of single crystal silicon solar cells. At the same time, large-area PSC modules have also developed rapidly. During the past few years, a lot of attempts have been conducted on large-scale manufacturing processes and perovskite precursor ink engineering, resulting in rapid increase in the efficiency of PSC modules, e.g., mini-module with a PCE of 16.4% and an aperture area 63.7 cm2 has been demonstrated [204]. However, there are still many issues for accelerating the commercialization of solution- processed PSCs, such as stability, toxicity and large-scale fabrication.
The stability issue of PSCs is one of the important factors hindering the commercialization. Although the stability of PSCs has been improved from several minutes to over one year, its improvement is not good enough for practical applications, which is still far away as compared with silicon solar cells. In order to enhance the stability of PSCs, systematic engineering including perovskite materials, charge transport materials, electrode material, structure design and encapsulation techniques should be considered. For example, the addition of two-dimensional perovskite has significantly improved the stability of PSCs. In particular, encapsulation plays a vital role in improving the stability and would help to accelerate the commercialization of PSCs.
As far as the toxicity of lead-based perovskite is concerned, researchers are trying to search for lead-free materials with similar optoelectronic properties. With theoretical calculations and experimental characterizations, a series of ions, like Sn2+,Ge2+, Cu2+, Bi3+, Sb3+, etc., in ABX3 structure can replace Pb2+ to form lead-free perovskites. Excitingly, organic-inorganic tin-based PSCs have now achieved PCE more than 10%, and there is still much room for improvement. The stability of Sn-based PSCs is far away from the requirement of commercialization. The origin of the instability is that facile oxidation of Sn2+ to Sn4+ and can decompose after exposure to water in air like the lead-based perovskite. So, the other huge challenge is how to restrain the oxidation of Sn2+ and improve the air stability of Sn-based perovskite materials.
PSCs fabricated by scalable printing and coating technologies have been impressive progress in the last few years. The control on nucleation and crystal growth of perovskite film are the key developments for the rapid progress in coated and printed large-area PSCs. The ink engineering allows a slow drying and processing time window for subsequent non-solvent treatment appears to be a promising route for reproduce the high-quality perovskite films in a variety large-scale printing or coating techniques, especially for R2R process. The in-situ and post-treatments, such as gas quenching, vacuum induced crystallization, elevated substrate temperatures, etc., can further enhance the quality of large-area perovskite films and accordingly highly efficient large-area PSCs. It is believed that the PCEs of scalable large-area PSCs or modules fabricated via solution-processed coating or printing techniques will be much closed to the ones fabricated via spin coating deposition. It is also the key point of future development of PSCs.
References
[1] GRATZEL M. The light and shade of perovskite solar cells [J]. Nature Materials, 2014, 13(9): 838-842. DOI: 10.1038/nmat4065.
[2] WEHRENFENNIG C, EPERON G E, JOHNSTON M B, SNAITH H J, HERZ L M. High charge carrier mobilities and lifetimes in organolead trihalide perovskites [J]. Advanced Materials, 2014, 26(10): 1584-1589. DOI: 10.1002/adma. 201305172.
[3] CHEN Qi, ZHOU Huan-ping, FANG Yi-hao, STIEG A Z, SONG T B, WANG H H, XU Xiao-bao, LIU Yong-sheng, LU Shi-rong, YOU Jing-bi, SUN Peng-yu, MCKAY J, GOORSKY M S, YANG Yang. The optoelectronic role of chlorine in CH3NH3PbI3(Cl)-based perovskite solar cells [J]. Nature Communications, 2015, 6(1): 1-9. DOI: 10.1038/ ncomms8269.
[4] XING Gui-chuan, MATHEWS N, SUN Shuang-yong, LIM S S, LAM Y M, GRATZEL M, MHAISALKAR S, SUM T C. Long-range balanced electron- and hole-transport lengths in organic-inorganic CH3NH3PbI3 [J]. Science, 2013, 342(6156): 344-347. DOI: 10.1126/science.1243167.
[5] DONG Qing-feng, FANG Yan-jun, SHAO Yu-chuan, MULLIGAN P, QIU Jie, CAO Lei, HUANG Jin-song. Electron-hole diffusion lengths >175 μm in solution-grown CH3NH3PbI3 single crystals [J]. Science, 2015, 347(6225): 967-970. DOI: 10.1126/science.aaa5760.
[6] NOH J H, IM S H, HEO J H, MANDAL T N, SEOK S II. Chemical management for colorful, efficient, and stable inorganic–organic hybrid nanostructured solar cells [J]. Nano Letters, 2013, 13(4): 1764-1769. DOI: 10.1021/nl400349b.
[7] JEON N J, NOH J H, YANG W S, KIM Y C, RYU S, SEO J, SEOK S II. Compositional engineering of perovskite materials for high-performance solar cells [J]. Nature, 2015, 517(7535): 476-480. DOI: 10.1038/nature14133.
[8] LIN Qian-qian, ARMIN A, NAGIRI R C R, BURN P L, MEREDITH P. Electro-optics of perovskite solar cells [J]. Nature Photonics, 2015, 9(2): 106-112. DOI: 10.1038/ nphoton.2014.284.
[9] YANG Ye, YANG Meng-jin, MOORE D T, YAN Yong, MILLER E M, ZHU Kai, BEARD M C. Top and bottom surfaces limit carrier lifetime in lead iodide perovskite films [J]. Nature Energy, 2017, 2(2): 1-7. DOI: 10.1038/nenergy. 2016.207.
[10] KOJIMA A, TESHIMA K, SHIRAI Y, MIYASAKA T. Organometal halide perovskites as visible-light sensitizers for photovoltaic cells [J]. Journal of the American Chemical Society, 2009, 131(17): 6050-6051. DOI: 10.1021/ ja809598r.
[11] ETGAR L, GAO Peng, XUE Zhao-sheng, PENG Qin, CHANDIRAN A K, LIU Bin, NAZEERUDDIN M K, GRATZEL M. Mesoscopic CH3NH3PbI3/TiO2 heterojunction solar cells [J]. Journal of the American Chemical Society, 2012, 134(42): 17396-17399. DOI: 10.1021/ja307789s.
[12] JIANG Qi, ZHAO Yang, ZHANG Xing-wang, YANG Xiao-lei, CHEN Yong, CHU Ze-ma, YE Qiu-feng, LI Xing-xing, YIN Zhi-gang, YOU Jing-bi. Surface passivation of perovskite film for efficient solar cells [J]. Nature Photonics, 2019, 13(4): 460-466. DOI: 10.1038/s41566- 019-0398-2.
[13] KIM Min-Jin, KIM Gi-Hwan, LEE Tae-Kyung, CHOI In-Woo, CHOI Hye-Won, JO Yim-Hyun, YOON Yung-Jin, KIM Jae-Won, LEE Ji-Yun, HUH Dai-Hong, LEE He-On, KWAK Sang-Kyu, KIM Jin-Young, KIM Dong-Suk. Methylammonium chloride induces intermediate phase stabilization for efficient perovskite solar cells [J]. Joule, 2019, 3(9): 2179-2192. DOI: 10.1016/j.joule.2019.06.014.
[14] YOO J J, WIEGHOLD S, SPONSELLER M C, CHUA M R, BERTRAM S N, HARTONO N T P, TRESBACK J S, HANSEN E C, CORREA-BAENA J P, BULOVIC V, BUONASSISI T, SHIN S S, BAWENDI M G. An interface stabilized perovskite solar cell with high stabilized efficiency and low voltage loss [J]. Energy & Environmental Science, 2019, 12(7): 2192-2199. DOI: 10.1039/C9EE00751B.
[15] JEON N J, NA H J, JUNG E H, YANG T Y, LEE Y G, KIM G J, SHIN H W, SEOK S II, LEE J M, SEO J W. A fluorene-terminated hole-transporting material for highly efficient and stable perovskite solar cells [J]. Nature Energy, 2018, 3(8): 682-689. DOI: 10.1038/s41560-018-0200-6.
[16] JUNG E H, JEON N J, PARK E Y, MOON C S, SHIN T J, YANG T Y, NOH J H, SEO J W. Efficient, stable and scalable perovskite solar cells using poly(3-hexylthiophene) [J]. Nature, 2019, 567(7749): 511-515. DOI: 10.1038/ s41586-019-1036-3.
[17] Photovoltaic Research, NREL. Best research-cell efficiency chart [EB/OL] [2019-10-24]. https://www.nrel.gov/pv/cell- efficiency.html.
[18] HOWARD I A, ABZIEHER T, HOSSAIN I M, EGGERS H, SCHACKMAR F, TERNES S, RICHARDS B S, LEMMER U, PAETZOLD U W. Coated and printed perovskites for photovoltaic applications [J]. Advanced Materials, 2019, 31(26): 1806702. DOI: 10.1002/adma.201806702.
[19] LI Zhen, KLEIN T R, KIM D H, YANG Meng-jin, BERRY J J, VAN HEST M F A M, ZHU Kai. Scalable fabrication of perovskite solar cells [J]. Nature Reviews Materials, 2018, 3(4): 18017. DOI: 10.1038/natrevmats.2018.17.
[20] SWARTWOUT R, HOERANTNER M T, BULOVIC V. Scalable deposition methods for large-area production of perovskite thin films [J]. Energy & Encironmental Materials, 2019, 2(2): 119-145. DOI: 10.1002/eem2.12043.
[21] WANG Peng, WU Yi-hui, CAI Bing, MA Qing-shan, ZHENG Xiao-jia, ZHANG Wen-hua. Solution-processable perovskite solar cells toward commercialization: Progress and challenges [J]. Advanced Functional Materials, 2019, 29(47): 1807661. DOI: 10.1002/adfm.201807661.
[22] YOU Jing-bi, HONG Zi-ruo, YANG Yang (Michael), CHEN Qi, CAI Min, SONG T B, CHEN C C, LU Shi-rong, LIU Yong-sheng, ZHOU Huan-ping, YANG Yang. Low-temperature solution-processed perovskite solar cells with high efficiency and flexibility [J]. ACS Nano, 2014, 8(2): 1674-1680. DOI: 10.1021/nn406020d.
[23] YOU Jing-bi, YANG Yang (Michael), HONG Zi-ruo, SONG T B, MENG Lei, LIU Yong-sheng, JIANG Cheng-yang, ZHOU Huan-ping, CHANG W H, LI Gang, YANG Yang. Moisture assisted perovskite film growth for high performance solar cells [J]. Applied Physics Letters, 2014, 105(18): 183902. DOI: 10.1063/1.4901510.
[24] MENG Lei, YOU Jing-bi, GUO Tzung-Fang, YANG Yang. Recent advances in the inverted planar structure of perovskite solar cells [J]. Accounts of Chemical Research, 2016, 49(1): 155-165. DOI: 10.1021/acs.accounts.5b00404.
[25] DOCAMPO P, BALL J M, DARWICH M, EPERON G E, SNAITH H J. Efficient organometal trihalide perovskite planar-heterojunction solar cells on flexible polymer substrates [J]. Nature Communications, 2013, 4(1): 1-6. DOI: 10.1038/ncomms3761.
[26] BURSCHKA J, PELLET N, MOON S J, HUMPHRY- BAKER R, GAO Peng, NAZEERUDDIN M K, GRATZEL M. Sequential deposition as a route to high-performance perovskite-sensitized solar cells [J]. Nature, 2013, 499(7458): 316-319. DOI: 10.1038/nature12340.
[27] SALIBA M, MATSUI T, DOMANSKI K, SEO J Y, UMMADISINGU A, ZAKEERUDDIN S M, CORREA-BAENA J P, TRESS W R, ABATE A, HAGFELDT A, GRATZEL M. Incorporation of rubidium cations into perovskite solar cells improves photovoltaic performance [J]. Science, 2016, 354(6309): 206-209. DOI: 10.1126/science.aah5557.
[28] MEI An-yi, LI Xiong, LIU Lin-feng, KU Zhi-liang, LIU Tong-fa, RONG Yao-guang, XU Mi, HU Min, CHEN Jiang-zhao, YANG Ying, GRATZEL M, HAN Hong-wei. A hole-conductor–free, fully printable mesoscopic perovskite solar cell with high stability [J]. Science, 2014, 345(6194): 295-298. DOI: 10.1126/science.1254763.
[29] XU Liao, WAN Fang, RONG Yao-guang, CHEN Hui, HE Song, XU Xiao-mei, LIU Gang, HAN Hong-wei, YUAN Yong-bo, YANG Jun-liang, GAO Yong-li, YANG Bing-chu, ZHOU Cong-hua. Stable monolithic hole-conductor-free perovskite solar cells using TiO2 nanoparticle binding carbon films [J]. Organic Electronics, 2017, 45: 131-138. DOI: 10.1016/j.orgel.2017.03.005.
[30] CHEN Hui, LI Kang-ming, LIU Huang, GAO Yong-li, YUAN Yong-bo, YANG Bing-chu, ZHOU Cong-hua. Dependence of power conversion properties of hole-conductor-free mesoscopic perovskite solar cells on the loading of perovskite crystallites [J]. Organic Electronics, 2018, 61: 119-124. DOI: 10.1016/j.orgel.2018.06.054.
[31] LI Kang-ming, CHEN Hui, LIU Huang, YUAN Yong-bo, GAO Yong-li, YANG Bing-chu, ZHOU Cong-hua. Dependence of power conversion properties of the hole-conductor-free mesoscopic perovskite solar cells on the thickness of carbon film [J]. Organic Electronics, 2018, 62: 298-303. DOI: 10.1016/j.orgel.2018.08.013.
[32] LIU Huang, YANG Bing-chu, CHEN Hui, LI Kang-ming, LIU Gang, YUAN Yong-bo, GAO Yong-li, ZHOU Cong-hua. Efficient and stable hole-conductor-free mesoscopic perovskite solar cells using SiO2 as blocking layer [J]. Organic Electronics, 2018, 58: 69-74. DOI: 10.1016/ j.orgel.2018.04.008.
[33] GIORDANO F, ABATE A, CORREA BAENA J P, SALIBA M, MATSUI T, IM S H, ZAKEERUDDIN S M, NAZEERUDDIN M K, HAGFELDT A, GRAETZEL M. Enhanced electronic properties in mesoporous TiO2 via lithium doping for high-efficiency perovskite solar cells [J]. Nature Communications, 2016, 7(1): 1-6. DOI: 10.1038/ ncomms10379.
[34] KIM D H, HAN G S, SEONG W M, LEE J W, KIM B J, PARK N G, HONG K S, LEE S W, JUNG H S. Niobium doping effects on TiO2 mesoscopic electron transport layer-based perovskite solar cells [J]. Chem Sus Chem, 2015, 8(14): 2392-2398. DOI: 10.1002/cssc.201403478.
[35] LIU Jian, WU Yong-zhen, QIN Chuan-jiang, YANG Xu-dong, YASUDA T, ISLAM A, ZHANG Kun, PENG Wen-qin, CHEN Wei, HAN Li-yuan. A dopant-free hole-transporting material for efficient and stable perovskite solar cells [J]. Energy & Environmental Science, 2014, 7(9): 2963-2967. DOI: 10.1039/C4EE01589D.
[36] SIDHIK S, CERDAN PASARAN A, ESPARZA D, LOPEZ LUKE T, CARRILES R, de LA ROSA E. Improving the optoelectronic properties of mesoporous TiO2 by cobalt doping for high-performance hysteresis-free perovskite solar cells [J]. ACS Applied Materials & Interfaces, 2018, 10(4): 3571-3580. DOI: 10.1021/acsami.7b16312.
[37] ZHAO Chen, CHEN Bing-bing, QIAO Xian-feng, LUAN Lin, LU Kai, HU Bin. Revealing underlying processes involved in light soaking effects and hysteresis phenomena in perovskite solar cells [J]. Advanced Energy Materials, 2015, 5(14): 1500279. DOI: 10.1002/aenm.201500279.
[38] LEE M M, TEUSCHER J, MIYASAKA T, MURAKAMI T N, SNAITH H J. Efficient hybrid solar cells based on meso-superstructured organometal halide perovskites [J]. Science, 2012, 338(6107): 643-647. DOI: 10.1126/science. 1228604.
[39] JIANG Qi, ZHANG Xing-wang, YOU Jing-bi. SnO2: A wonderful electron transport layer for perovskite solar cells [J]. Small, 2018, 14(31): 1801154. DOI: 10.1002/smll. 201801154.
[40] EPERON G E, BURLAKOV V M, DOCAMPO P, GORIELY A, SNAITH H J. Morphological control for high performance, solution-processed planar heterojunction perovskite solar cells [J]. Advanced Functional Materials, 2014, 24(1): 151-157. DOI: 10.1002/adfm.201302090.
[41] EPERON G E, STRANKS S D, MENELAOU C, JOHNSTON M B, HERZ L M, SNAITH H J. Formamidinium lead trihalide: A broadly tunable perovskite for efficient planar heterojunction solar cells [J]. Energy & Environmental Science, 2014, 7(3): 982-988. DOI: 10.1039/C3EE43822H.
[42] HEO J H, SONG D H, HAN H J, KIM S Y, KIM J H, KIM D, SHIN H W, AHN T K, WOLF C, LEE T W, IM S H. Planar CH3NH3PbI3 perovskite solar cells with constant 17.2% average power conversion efficiency irrespective of the scan rate [J]. Advanced Materials, 2015, 27(22): 3424-3430. DOI: 10.1002/adma.201500048.
[43] XU Ji-xian, BUIN A, IP A H, LI Wei, VOZNYY O, COMIN R, YUAN Ming-jian, JEON S, NING Zhi-jun, MCDOWELL J J, KANJANABOOS P, SUN J P, LAN Xin-zheng, QUAN Li-na, KIM D H, HILL I G, MAKSYMOVYCH P, SARGENT E H. Perovskite–fullerene hybrid materials suppress hysteresis in planar diodes [J]. Nature Communications, 2015, 6(1): 1-8. DOI: 10.1038/ ncomms8081.
[44] LI Liang, CHEN Yi-hua, LIU Zong-hao, CHEN Qi, WANG Xin-dong, ZHOU Huan-ping. The additive coordination effect on hybrids perovskite crystallization and high- performance solar cell [J]. Advanced Materials, 2016, 28(44): 9862-9868. DOI: 10.1002/adma.201603021.
[45] LI Shao-sian, CHANG Chi-huang, WANG Ying-chiao, LIN Chung-wei, WANG Di-yan, LIN J C, CHEN C C, SHEU H S, CHIA Hao-chung, WU Wei-ru, JENG U S, LIANG Chi-te, SANKAR R, CHOU Fang-cheng, CHEN Chun-wei. Intermixing-seeded growth for high-performance planar heterojunction perovskite solar cells assisted by precursor- capped nanoparticles [J]. Energy & Environmental Science, 2016, 9(4): 1282-1289. DOI: 10.1039/C5EE03229F.
[46] JENG Jun-yuan, CHIANG Yi-fang, LEE Mu-huan, PENG Shin-rung, GUO Tzung-fang, CHEN P, WEN Ten-chin. CH3NH3PbI3 perovskite/fullerene planar-heterojunction hybrid solar cells [J]. Advanced Materials, 2013, 25(27): 3727-3732. DOI: 10.1002/adma.201301327.
[47] HYUCKH J, JIHAN H, KIM D, KYUAHN T, HYUKI M S. Hysteresis-less inverted CH3NH3PbI3 planar perovskite hybrid solar cells with 18.1% power conversion efficiency [J]. Energy & Environmental Science, 2015, 8(5): 1602-1608. DOI: 10.1039/C5EE00120J.
[48] TURREN-CRUZ S H, SALIBA M, MAYER M T, JUAREZ-SANTIESTEBAN H, MATHEW X, NIENHAUS L, TRESS W, ERODICI M P, SHER M J, BAWENDI M G, GRATZEL M, ABATE A, HAGFELDT A, CORREA- BAENA J P. Enhanced charge carrier mobility and lifetime suppress hysteresis and improve efficiency in planar perovskite solar cells [J]. Energy & Environmental Science, 2018, 11(1): 78-86. DOI: 10.1039/C7EE02901B.
[49] WANG Qi, SHAO Yu-chuan, DONG Qing-feng, XIAO Zheng-guo, YUAN Yong-bo, HUANG Jin-song. Large fill-factor bilayer iodine perovskite solar cells fabricated by a low-temperature solution-process [J]. Energy & Environmental Science, 2014, 7(7): 2359-2365. DOI: 10.1039/C4EE00233D.
[50] BI D, MOON S J, HAGGMAN L, BOSCHLOO G, YANG Lei, JOHANSSON E M J, NAZEERUDDIN M K, GRATZEL M, HAGFELDT A. Using a two-step deposition technique to prepare perovskite (CH3NH3PbI3) for thin film solar cells based on ZrO2 and TiO2 mesostructures [J]. RSC Advances, 2013, 3(41): 18762. DOI: 10.1039/c3ra43228a.
[51] CHEN Qi, ZHOU Huan-ping, HONG Zi-ruo, LUO Song, DUAN Hsin-sheng, WANG Hsin-hua, LIU Yong-sheng, LI Gang, YANG Yang. Planar heterojunction perovskite solar cells via vapor-assisted solution process [J]. Journal of the American Chemical Society, 2014, 136(2): 622-625. DOI: 10.1021/ja411509g.
[52] DOCAMPO P, HANUSCH F C, STRANKS S D, DOBLINGER M, FECKL J M, EHRENSPERGER M, MINAR N K, JOHNSTON M B, SNAITH H J, BEIN T. Solution deposition-conversion for planar heterojunction mixed halide perovskite solar cells [J]. Advanced Energy Materials, 2014, 4(14): 1400355. DOI: 10.1002/aenm. 201400355.
[53] XIAO Zheng-guo, BI Cheng, SHAO Yu-chuan, DONG Qing-feng, WANG Qi, YUAN Yong-bo, WANG Cheng-gong, GAO Yong-li, HUANG Jin-song. Efficient, high yield perovskite photovoltaic devices grown by interdiffusion of solution-processed precursor stacking layers [J]. Energy & Environmental Science, 2014, 7(8): 2619-2623. DOI: 10.1039/C4EE01138D.
[54] BI Cheng, WANG Qi, SHAO Yu-chuan, YUAN Yong-bo, XIAO Zheng-guo, HUANG Jin-song. Non-wetting surface- driven high-aspect-ratio crystalline grain growth for efficient hybrid perovskite solar cells [J]. Nature Communications, 2015, 6(1): 7747. DOI: 10.1038/ncomms8747.
[55] WANG Qi, DONG Qing-feng, LI Tao, GRUVERMAN A, HUANG Jin-song. Thin insulating tunneling contacts for efficient and water-resistant perovskite solar cells [J]. Advanced Materials, 2016, 28(31): 6734-6739. DOI: 10.1002/adma.201600969.
[56] KIM J H, WILLIAMS S T, CHO N, CHUEH C C, JEN A K Y. Enhanced environmental stability of planar heterojunction perovskite solar cells based on blade-coating [J]. Advanced Energy Materials, 2015, 5(4): 1401229. DOI: 10.1002/ aenm.201401229.
[57] BARROWS A T, PEARSON A J, KWAK C K, DUNBAR A D F, BUCKLEY A R, LIDZEY D G. Efficient planar heterojunction mixed-halide perovskite solar cells deposited via spray-deposition [J]. Energy & Environmental Science, 2014, 7(9): 2944-2950. DOI: 10.1039/C4EE01546K.
[58] VAK D, HWANG K, FAULKS A, JUNG Y S, CLARK N, KIM D Y, WILSON G J, WATKINS S E. 3D printer-based slot-die coater as a lab-to-fab translation tool for solution-processed solar cells [J]. Advanced Energy Materials, 2015, 5(4): 1401539. DOI: 10.1002/aenm. 201401539
[59] WU Han, ZHANG Chu-jun, DING Kong-xian, WANG Li-juan, GAO Yong-li, YANG Jun-liang. Efficient planar heterojunction perovskite solar cells fabricated by in-situ thermal-annealing doctor blading in ambient condition [J]. Organic Electronics, 2017, 45: 302-307. DOI: 10.1016/ j.orgel.2017.03.017.
[60] PENG Yong-yi, CHENG Yu-diao, WANG Chun-hua, ZHANG Chu-jun, XIA Hua-yan, HUANG Ke-qing, TONG Si-chao, HAO Xiao-tao, YANG Jun-liang. Fully doctor- bladed planar heterojunction perovskite solar cells under ambient condition [J]. Organic Electronics, 2018, 58: 153-158. DOI: 10.1016/j.orgel.2018.04.020.
[61] HU Qiao, WU Han, SUN Jia, YAN Dong-hang, GAO Yong-li, YANG Jun-liang. Large-area perovskite nanowire arrays fabricated by large-scale roll-to-roll micro-gravure printing and doctor blading [J]. Nanoscale, 2016, 8(9): 5350-5357. DOI: 10.1039/C5NR08277C.
[62] LI Heng-yue, GUO Hui, TONG Si-chao, HUANG Ke-qing, ZHANG Chu-jun, WANG Xiao-feng, ZHANG Dou, CHEN Xiao-hua, YANG Jun-liang. High-performance supercapacitor carbon electrode fabricated by large-scale roll-to-roll micro-gravure printing [J]. Journal of Physics D: Applied Physics, 2019, 52(11): 115501. DOI: 10.1088/ 1361-6463/aafbf3.
[63] TONG Si-chao, WU Han, ZHANG Chu-jun, LI Shui-gen, WANG Chun-hua, SHEN Jian-qiang, XIAO Si, HE Jun, YANG Jun-liang, SUN Jia, GAO Yong-li. Large-area and high-performance CH3NH3PbI3 perovskite photodetectors fabricated via doctor blading in ambient condition [J]. Organic Electronics, 2017, 49: 347-354. DOI: 10.1016/ j.orgel.2017.07.011.
[64] DENG Ye-hao, ZHENG Xiao-peng, BAI Yang, WANG Qi, ZHAO Jing-jing, HUANG Jin-song. Surfactant-controlled ink drying enables high-speed deposition of perovskite films for efficient photovoltaic modules [J]. Nature Energy, 2018, 3(7): 560-566. DOI: 10.1038/s41560-018-0153-9.
[65] DENG Ye-hao, DONG Qing-feng, BI Cheng, YUAN Yong-bo, HUANG Jin-song. Air-stable, efficient mixed- cation perovskite solar cells with Cu electrode by scalable fabrication of active layer [J]. Advanced Energy Materials, 2016, 6(11): 1600372. DOI: 10.1002/aenm.201600372.
[66] TANG Shi, DENG Ye-hao, ZHENG Xiao-peng, BAI Yang, FANG Yan-jun, DONG Qing-feng, WEI Hao-tong, HUANG Jin-song. Composition engineering in doctor-blading of perovskite solar cells [J]. Advanced Energy Materials, 2017, 7(18): 1700302. DOI: 10.1002/aenm.201700302.
[67] WU Wu-qiang, YANG Zhi-bin, RUDD P N, SHAO Yu-chuan, DAI Xue-zeng, WEI Hao-tong, ZHAO Jing-jing, FANG Yan-jun, WANG Qi, LIU Ye, DENG Ye-hao, XIAO Xun, FENG Yuan-xiang, HUANG Jin-song. Bilateral alkylamine for suppressing charge recombination and improving stability in blade-coated perovskite solar cells [J]. Science Advances, 2019, 5(3): eaav8925. DOI: 10.1126/ sciadv.aav8925.
[68] WU Wu-qiang, WANG Qi, FANG Yan-jun, SHAO Yu-chuan, TANG Shi, DENG Ye-hao, LU Hai-dong, LIU Ye, LI Tao, YANG Zhi-bin, GRUVERMAN A, HUANG Jin-song. Molecular doping enabled scalable blading of efficient hole-transport-layer-free perovskite solar cells [J]. Nature Communications, 2018, 9(1): 1625. DOI: 10.1038/s41467- 018-04028-8.
[69] JUNG M, JI S G, KIM G, SEOK S Ⅱ. Perovskite precursor solution chemistry: From fundamentals to photovoltaic applications [J]. Chemical Society Reviews, 2019, 48(7): 2011-2038. DOI: 10.1039/C8CS00656C.
[70] HWANG K, JUNG Y S, HEO Y J, SCHOLES F H, WATKINS S E, SUBBIAH J, JONES D J, KIM D Y, VAK D. Toward large scale roll-to-roll production of fully printed perovskite solar cells[J]. Advanced Materials, 2015, 27(7): 1241-1247. DOI: 10.1002/adma.201404598.
[71] WHITAKER J B, KIM D H, LARSON BW, ZHANG Fei, BERRY J J, VAN HEST M F A M, ZHU Kai. Scalable slot-die coating of high-performance perovskite solar cells [J]. Sustainable Energy & Fuels, 2018, 2(11): 2442-2449. DOI: 10.1039/C8SE00368H.
[72] GONG Chen-di, TONG Si-chao, HUANG Ke-qing, LI Heng-yue, HUANG Han, ZHANG Jian, YANG Jun-liang. Flexible planar heterojunction perovskite solar cells fabricated via sequential roll-to-roll microgravure printing and slot-die coating deposition [J]. Solar RRL, 2019: 1900204. DOI: 10.1002/solr.201900204.
[73] HEO J H, LEE M H, JANG M H, IM S H. Highly efficient CH3NH3PbI3-xCl mixed halide perovskite solar cells prepared by re-dissolution and crystal grain growth via spray coating [J]. Journal of Materials Chemistry A, 2016, 4(45): 17636-17642. DOI: 10.1039/C6TA06718B.
[74] ZHANG Chu-jun, LUO Qun, WU Han, LI Heng-yue, LAI Jun-qi, JI Guo-qi, YAN Lin-peng, WANG Xiao-feng, ZHANG Dou, LIN Jian, CHEN Li-wei, YANG Jun-liang, MA Chang-qi. Roll-to-roll micro-gravure printed large-area zinc oxide thin film as the electron transport layer for solution-processed polymer solar cells [J]. Organic Electronics, 2017, 45: 190-197. DOI: 10.1016/j.orgel.2017. 03.015.
[75] YANG Jun-liang, VAK D, CLARK N, SUBBIAH J, WONG W W H, JONES D J, WATKINS S E, WILSON G. Organic photovoltaic modules fabricated by an industrial gravure printing proofer [J]. Solar Energy Materials and Solar Cells, 2013, 109: 47-55. DOI: 10.1016/j.solmat.2012.10.018.
[76] TONG Si-chao, GONG Chen-di, ZHANG Chu-jun, LIU Gang, ZHANG Dou, ZHOU Cong-hua, SUN Jia, XIAO Si, HE Jun, GAO Yong-li, YANG Jun-liang. Fully-printed, flexible cesium-doped triple cation perovskite photodetector [J]. Applied Materials Today, 2019, 15: 389-397. DOI: 10.1016/j.apmt.2019.03.001.
[77] LI Shao-gang, JIANG Ke-jian, SU Mei-ju, CUI Xue-ping, HUANG Jin-hua, ZHANG Qian-qian, ZHOU Xue-qin, YANG Lian-min, SONG Yan-lin. Inkjet printing of CH3NH3PbI3 on a mesoscopic TiO2 film for highly efficient perovskite solar cells [J]. Journal of Materials Chemistry A, 2015, 3(17): 9092-9097. DOI: 10.1039/C4TA05675B.
[78] EGGERS H, SCHACKMAR F, ABZIEHER T, SUN Qing, LEMMER U, VAYNZOF Y, RICHARDS B S, HERNANDEZ-SOSA G, PAETZOLD U W. Inkjet-printed micrometer-thick perovskite solar cells with large columnar grains [J]. Advanced Energy Materials, 2019, 9: 1903184. DOI: 10.1002/aenm.201903184.
[79] RAZZA S, CASTRO-HERMOSA S, DI CARLO A, BROWN T M. Research update: Large-area deposition, coating, printing, and processing techniques for the upscaling of perovskite solar cell technology [J]. APL Materials, 2016, 4(9): 091508. DOI: 10.1063/1.4962478.
[80] HE Ming, LI Bo, CUI Xun, JIANG Bei-bei, HE Yan-jie, CHEN Yi-huang, O’NEIL D, SZYMANSKI P, EI-SAYED M A, HUANG Jin-song, LIN Zhi-qun. Meniscus-assisted solution printing of large-grained perovskite films for high-efficiency solar cells [J]. Nature Communications, 2017, 8(1): 16045. DOI: 10.1038/ncomms16045.
[81] NIE W, TSAI H, ASADPOUR R, BLANCON J C, NEUKIRCH A J, GUPTA G, CROCHET J J, CHHOWALLA M, TRETIAK S, ALAM M A, WANG H L, MOHITE A D. High-efficiency solution-processed perovskite solar cells with millimeter-scale grains [J]. Science, 2015, 347(6221): 522-525. DOI: 10.1126/science. aaa0472.
[82] YE Fei, CHEN Han, XIE Feng-xian, TANG Wen-tao, YIN Mao-shu, HE Jin-jin, BI En-bing, WANG Yan-bo, YANG Xu-dong, HAN Li-yuan. Soft-cover deposition of scaling-up uniform perovskite thin films for high cost-performance solar cells [J]. Energy & Environmental Science, 2016, 9(7): 2295-2301. DOI: 10.1039/C6EE01411A.
[83] CAI Mo-lang, WU Yong-zhen, CHEN Han, YANG Xu-dong, QIANG Ying-huai, HAN Li-yuan. Cost-performance analysis of perovskite solar modules [J]. Advanced Science, 2017, 4(1): 1600269. DOI: 10.1002/advs.201600269.
[84] XIAO Zheng-guo, DONG Qing-feng, BI Cheng, SHAO Yu-chuan, YUAN Yong-bo, HUANG Jin-song. Solvent annealing of perovskite-induced crystal growth for photovoltaic-device efficiency enhancement [J]. Advanced Materials, 2014, 26(37): 6503-6509. DOI: 10.1002/adma. 201401685.
[85] BI Cheng, YUAN Yong-bo, FANG Yan-jun, HUANG Jin-song. Low-temperature fabrication of efficient wide-bandgap organolead trihalide perovskite solar cells [J]. Advanced Energy Materials, 2015, 5(6): 1401616. DOI: 10.1002/aenm.201401616.
[86] JEON N J, NOH J H, KIM Y C, YANG W S, RYU S, SEOK S Ⅱ. Solvent engineering for high-performance inorganic–organic hybrid perovskite solar cells [J]. Nature Materials, 2014, 13(9): 897-903. DOI: 10.1038/nmat4014.
[87] NG A, REN Zhi-wei, HU Han-lin, FONG P W K, SHEN Qian, CHEUNG S H, QIN Ping-li, LEE J W, DJURISIC A B, SO S K, LI Gang, YANG Yang, SURYA C. A cryogenic process for antisolvent-free high-performance perovskite solar cells [J]. Advanced Materials, 2018, 30(44): 1804402. DOI: 10.1002/adma.201804402.
[88] XUE Jing-jing, WANG Rui, WANG Kai-li, WANG Zhao-kui, YAVUZ I, WANG Yang, YANG Ying-guo, GAO Xing-yu, HUANG Tian-yi, NURYYEVA S, LEE J W, DUAN Yu, LIAO Liang-sheng, KANER R, YANG Yang. Crystalline liquid-like behavior: surface-induced secondary grain growth of photovoltaic perovskite thin film [J]. Journal of the American Chemical Society, 2019, 141(35): 13948-13953. DOI: 10.1021/jacs.9b06940.
[89] ZHOU Zhong-min, WANG Zai-wei, ZHOU Yuan-yuan, PANG Shu-ping, WANG Dong, XU Hong-xia, LIU Zhi-hong, PADTURE N P, CUI Guang-lei. Methylamine- gas-induced defect-healing behavior of CH3NH3PbI3 thin films for perovskite solar cells [J]. Angewandte Chemie International Edition, 2015, 54(33): 9705-9709. DOI: 10.1002/anie.201504379.
[90] YANG Meng-jin, ZHANG Tai-yang, SCHULZ P, LI Zhen, LI Ge, KIM D H, GUO Nan-jie, BERRY J J, ZHU Kai, ZHAO Yi-xin. Facile fabrication of large-grain CH3NH3PbI3-xBrx films for high-efficiency solar cells via CH3NH3Br-selective Ostwald ripening [J]. Nature Communications, 2016, 7: 12305. DOI: 10.1038/ ncomms12305.
[91] SHAO Yu-chuan, XIAO Zheng-guo, BI Cheng, YUAN Yong-bo, HUANG Jin-song. Origin and elimination of photocurrent hysteresis by fullerene passivation in CH3NH3PbI3 planar heterojunction solar cells [J]. Nature Communications, 2014, 5(1): 5784. DOI: 10.1038/ ncomms6784.
[92] BAI Yang, DONG Qing-feng, SHAO Yu-chuan, DENG Ye-hao, WANG Qi, SHEN Liang, WANG Dong, WEI Wei, HUANG Jin-song. Enhancing stability and efficiency of perovskite solar cells with crosslinkable silane- functionalized and doped fullerene [J]. Nature Communications, 2016, 7(1): 12806. DOI: 10.1038/ ncomms12806.
[93] ZHENG Xiao-peng, CHEN Bo, DAI Jun, FANG Yan-jun, BAI Yang, LIN Yu-ze, WEI Hao-tong, ZENG Xiao-cheng, HUANG Jin-song. Defect passivation in hybrid perovskite solar cells using quaternary ammonium halide anions and cations [J]. Nature Energy, 2017, 2(7): 17102. DOI: 10.1038/nenergy.2017.102.
[94] LIN Yun, BAI Yang, FANG Yan-jun, CHEN Zhao-lai, YANG Shuang, ZHENG Xiao-peng, TANG Shi, LIU Ye, ZHAO Jing-jing, HUANG Jin-song. Enhanced thermal stability in perovskite solar cells by assembling 2D/3D stacking structures [J]. The Journal of Physical Chemistry Letters, 2018, 9(3): 654-658. DOI: 10.1021/acs.jpclett. 7b02679.
[95] HUEH C C, LI Chang-zhi,JEN A K Y. Recent progress and perspective in solution-processed interfacial materials for efficient and stable polymer and organometal perovskite solar cells [J]. Energy & Environmental Science, 2015, 8(4): 1160-1189. DOI: 10.1039/C4EE03824J.
[96] ZHOU Huan-ping, CHEN Qi, LI Gang, LUO Song, SONG Tze-bing, DUAN Hsin-sheng, HONG Zi-ruo, YOU Jing-bi, LIU Yong-sheng, YANG Yang. Interface engineering of highly efficient perovskite solar cells [J]. Science, 2014, 345(6196): 542-546. DOI: 10.1126/science.1254050.
[97] PATHAK S K, ABATE A, RUCKDESCHEL P, ROOSE B, GODEL K C, VAYNZOF Y, SANTHALA A, WATANABE S I, HOLLMAN D J, NOEL N, SEPE A, WIESNER U, FRIEND R, SNAITH H J, STEINER U. Performance and stability enhancement of dye-sensitized and perovskite solar cells by al doping of TiO2 [J]. Advanced Functional Materials, 2014, 24(38): 6046-6055. DOI: 10.1002/adfm. 201401658.
[98] LIU Zong-hao, HU Jun-nan, JIAO Hao-yang, LI Liang, ZHENG Guanhaojie, CHEN Yi-hua, HUANG Yuan, ZHANG Qing, SHEN Chao, CHEN Qi, ZHOU Huan-ping. Chemical reduction of intrinsic defects in thicker heterojunction planar perovskite solar cells [J]. Advanced Materials, 2017, 29(23): 1606774. DOI: 10.1002/adma. 201606774.
[99] TAN Hai-ren, JAIN A, VOZNYY O, LAN Xin-zheng, ARQUER F P G D, FAN J Z, QUINTERO-BERMUDEZ R, YUAN Ming-jian, ZHANG Bo, ZHAO Yi-cheng, FAN Feng-jia, LI Pei-cheng, QUAN Li-na, ZHAO Yong-biao, LU Zheng-hong, YANG Zhen-yu, HOOGLAND S, SARGENT E H. Efficient and stable solution-processed planar perovskite solar cells via contact passivation [J]. Science, 2017, 355(6326): 722-726. DOI: 10.1126/science.aai9081.
[100] UAN Zhong-cheng, WU Zhong-wei, BAI Sai, XIA Zhou-hui, XU Wei-dong, SONG Tao, WU Hai-hua, XU Lu-hai, SI Jun-jie, JIN Yi-zheng, SUN Bao-quan. Hot-electron injection in a sandwiched TiOx–Au–TiOx structure for high- performance planar perovskite solar cells [J]. Advanced Energy Materials, 2015, 5(10): 1500038. DOI: 10.1002/ aenm.201500038.
[101] TAVAKOLI M M, YADAV P, TAVAKOLI R, KONG Jing. Surface engineering of TiO2 ETL for highly efficient and hysteresis-less planar perovskite solar cell (21.4%) with enhanced open-circuit voltage and stability [J]. Advanced Energy Materials, 2018, 8(23): 1800794. DOI: 10.1002/ aenm.201800794.
[102] BAENA J P C, STEIER L, TRESS W, SALIBA M, NEUTZNER S, MATSUI T, GIORDANO F, JESPERJACOBSSON T, KANDADA A R S, M. ZAKEERUDDIN S, PETROZZA A, ABATE A, KHAJANAZEERUDDIN M, GRATZEL M, HAGFELDT A. Highly efficient planar perovskite solar cells through band alignment engineering [J]. Energy & Environmental Science, 2015, 8(10): 2928-2934. DOI: 10.1039/C5EE02608C.
[103] RAO H S, CHEN Bai-xue, LI Wen-guang, XU Yang-fan, CHEN Hong-yan, KUANG Dai-bin, SU Cheng-yong. Improving the extraction of photogenerated electrons with SnO2 nanocolloids for efficient planar perovskite solar cells [J]. Advanced Functional Materials, 2015, 25(46): 7200- 7207. DOI: 10.1002/adfm.201501264.
[104] HALVANIANARAKI E, KERMANPUR A, STEIER L, DOMANSKI K, MATSUI T, TRESS W, SALIBA M, ABATE A, GRATZEL M, HAGFELDT A, CORREA- BAENA J P. Highly efficient and stable planar perovskite solar cells by solution-processed tin oxide [J]. Energy & Environmental Science, 2016, 9(10): 3128-3134. DOI: 10.1039/C6EE02390H.
[105] WANG Chun-hua, ZHANG Chu-jun, WANG Shi-tan, LIU Gang, XIA Hua-yan, TONG Si-chao, HE Jun, NIU Dong-mei, ZHOU Cong-hua, DING Kong-xian, GAO Yong-li, YANG Jun-liang. Low-temperature processed, efficient, and highly reproducible cesium-doped triple cation perovskite planar heterojunction solar cells [J]. Solar RRL, 2018, 2(2): 1700209. DOI: 10.1002/solr.201700209.
[106] HUANG Ke-qing, PENG Yong-yi, GAO Ya-xin, SHI Jiao, LI Heng-yue, MO Xin-di, HUANG Han, GAO Yong-li, DING Li-ming, YANG Jun-liang. High-performance flexible perovskite solar cells via precise control of electron transport layer [J]. Advanced Energy Materials, 2019, 9(44): 1901419. DOI: 10.1002/aenm.201901419.
[107] HUANG Ke-qing, LI Heng-yue, ZHANG Chu-jun, GAO Ya-xin, LIU Tian-jiao, ZHANG Jian, GAO Yong-li, PENG Yong-yi, DING Li-ming, YANG Jun-liang. Highly efficient perovskite solar cells processed under ambient conditions using in situ substrate-heating-assisted deposition [J]. Solar RRL, 2019, 3(3): 1800318. DOI: 10.1002/solr.201800318.
[108] WANG Chun-hua, YANG Jun-liang. Interface modification for organic and perovskite solar cells [J]. Science China Materials, 2016, 59(9): 743-756. DOI: 10.1007/s40843-016- 5080-1.
[109] GAO Ya-xin, DONG Ya-nan, HUANG Ke-qing, ZHANG Chu-jun, LIU Biao, WANG Shi-tan, SHI Jiao, XIE Hai-peng, HUANG Han, XIAO Si, HE Jun, GAO Yong-li, HATTON R A, YANG Jun-liang. Highly efficient, solution-processed CsPbI2Br planar heterojunction perovskite solar cells via flash annealing [J]. ACS Photonics, 2018, 5(10): 4104-4110. DOI: 10.1021/acsphotonics.8b00783.
[110] JIANG Qi, ZHANG Liu-qi, WANG Hao-lin, YANG Xiao-lei, MENG Jun-hua, LIU Heng, YIN Zhi-gang, WU Jin-liang, ZHANG Xing-wang, YOU Jing-bi. Enhanced electron extraction using SnO2 for high-efficiency planar-structure HC(NH2)2PbI3-based perovskite solar cells [J]. Nature Energy, 2016, 2(1): 1-7. DOI: 10.1038/nenergy.2016.177.
[111] KE Wei-jun, ZHAO De-wei, XIAO Chuan-xiao, WANG Chang-lei, CIMAROLI A J, GRICE C R, YANG Meng-jin, LI Zhen, JIANG Chun-sheng, AL-JASSIM M, ZHU Kai, KANATZIDIS M G, FANG Guo-jia, YAN Yan-fa. Cooperative tin oxide fullerene electron selective layers for high-performance planar perovskite solar cells [J]. Journal of Materials Chemistry A, 2016, 4(37): 14276-14283. DOI: 10.1039/C6TA05095F.
[112] WU Wu-qiang, CHEN De-hong, CHENG Yi-bing, CARUSO R A. Thin films of tin oxide nanosheets used as the electron transporting layer for improved performance and ambient stability of perovskite photovoltaics [J]. Solar RRL, 2017, 1(11): 1700117. DOI: 10.1002/solr.201700117.
[113] YANG Dong, YANG Rui-xia, WANG Kai, WU Cong-cong, ZHU Xue-jie, FENG Jiang-shan, REN Xiao-dong, FANG Guo-jia, PRIYA S, LIU Sheng-zhong (Frank). High efficiency planar-type perovskite solar cells with negligible hysteresis using EDTA-complexed SnO2 [J]. Nature Communications, 2018, 9(1): 1-11. DOI: 10.1038/s41467- 018-05760-x.
[114] HU Man-man, ZHANG Luo-zheng, SHE Su-yang, WU Jian-chang, ZHOU Xian-yong, LI Xiang-nan, WANG Deng, MIAO Jun, MI Guo-jun, CHEN Hong, TIAN Yan-qing, XU Bao-min, CHENG Chun. Electron transporting bilayer of SnO2 and TiO2 nanocolloid enables highly efficient planar perovskite solar cells [J]. Solar RRL, 2019: 1900331. DOI: 10.1002/solr.201900331.
[115] HUI Wei, YANG Ying-guo, XU Quan, GU Hao, FENG Shang-lei, SU Zhen-huang, ZHANG Miao-ran, WANG Jiaou, LI Xiao-dong, FANG Jun-feng, XIA Fei, XIA Ying-dong, CHEN Yong-hua, GAO Xing-yu, HUANG Wei. Red-carbon-quantum-dot-doped SnO2 composite with enhanced electron mobility for efficient and stable perovskite solar cells [J]. Advanced Materials, 2019, 9: 1906374. DOI: 10.1002/adma.201906374.
[116] ZHU Peng-chen, GU Shuai, LUO Xin, GAO Yuan, LI Song-lin, ZHU Jia, TAN Hai-ren. Simultaneous contact and grain-boundary passivation in planar perovskite solar cells using SnO2-KCl composite electron transport layer [J]. Advanced Energy Materials, 2019, 10(3): 1903083. DOI: 10.1002/aenm.201903083.
[117] CHEN Jin-bo, DONG Hua, ZHANG Lin, LI Jing-rui, JIA Fu-hao, JIAO Bo, JIE Xu, HOU Xun, LIU Jian, WU Zhao-xin. Graphitic carbon nitride doped SnO2 enabling efficient perovskite solar cells exceeding 22% [J]. Journal of Materials Chemistry A, 2020. DOI: 10.1039/C9TA11344D.
[118] TIWANA P, DOCAMPO P, JOHNSTON M B, SNAITH H J, HERZ L M. Electron Mobility and injection dynamics in mesoporous ZnO, SnO2, and TiO2 films used in dye-sensitized solar cells [J]. ACS Nano, 2011, 5(6): 5158-5166. DOI: 10.1021/nn201243y.
[119] HEO J H,LEE M H, HAN H J,PATIL R B, YU J S,IM S H. Highly efficient low temperature solution processable planar type CH3NH3PbI3 perovskite flexible solar cells [J]. Journal of Materials Chemistry A, 2016, 4(5): 1572-1578. DOI: 10.1039/C5TA09520D.
[120] YANG Jin-li, SIEMPELKAMP B D, MOSCONI E, de ANGELIS F, KELLY T L. Origin of the thermal instability in CH3NH3PbI3 thin films deposited on ZnO [J]. Chemistry of Materials, 2015, 27(12): 4229-4236. DOI: 10.1021/acs. chemmater.5b01598.
[121] AZMI R, HWANG S, YIN Wen-ping, KIM T W, AHN T K, JANG S Y. High efficiency low-temperature processed perovskite solar cells integrated with alkali metal doped ZnO electron transport layers [J]. ACS Energy Letters, 2018, 3(6): 1241-1246. DOI: 10.1021/acsenergylett.8b00493.
[122] MAHDITAVAKOLI M, TAVAKOLI R, YADAV P, KONG Jing. A graphene/ZnO electron transfer layer together with perovskite passivation enables highly efficient and stable perovskite solar cells [J]. Journal of Materials Chemistry A, 2019, 7(2): 679-686. DOI: 10.1039/ C8TA10857A.
[123] QIN Min-chao, MA Jun-jie, KE Wei-jun, QIN Ping-li, LEI Hong-wei, TAO Hong, ZHENG Xiao-lu, XIONG Liang-bin, LIU Qin, CHEN Zhi-liang, LU Jun-zheng, YANG Guang, FANG Guo-jia. Perovskite solar cells based on low-temperature processed indium oxide electron selective layers [J]. ACS Applied Materials & Interfaces, 2016, 8(13): 8460-8466. DOI: 10.1021/acsami.5b12849.
[124] CHEN Peng, YIN Xing-tian, QUE Mei-dan, LIU Xiao-bin, QUE Wen-xiu. Low temperature solution processed indium oxide thin films with reliable photoelectrochemical stability for efficient and stable planar perovskite solar cells [J]. Journal of Materials Chemistry A, 2017, 5(20): 9641-9648. DOI: 10.1039/C7TA00183E.
[125] YOON S, KIM S J, KIM H S, PARK J S, KIHAN II K,JUNG J W, PARK M. Solution-processed indium oxide electron transporting layers for high-performance and photo-stable perovskite and organic solar cells [J]. Nanoscale, 2017, 9(42): 16305-16312. DOI: 10.1039/C7NR05695H.
[126] LING Xu-feng, YUAN Jian-yu, LIU Dong-yang, WANG Yong-jie, ZHANG Yan-nan, CHEN Si, WU Hai-hua, JIN Feng, WU Fu-peng, SHI Guo-zheng, TANG Xun, ZHENG Jia-wei, LIU Sheng-zhong (Frank), LIU Zhi-ke, MA Wan-li. Room-temperature processed Nb2O5 as the electron- transporting layer for efficient planar perovskite solar cells [J]. ACS Applied Materials & Interfaces, 2017, 9(27): 23181-23188. DOI: 10.1021/acsami.7b05113.
[127] WANG Zeng-hua, LOU Jun-jie, ZHENG Xiao-jia, ZHANG Wen-hua, QIN Yong. Solution processed Nb2O5 electrodes for high efficient ultraviolet light stable planar perovskite solar cells [J]. ACS Sustainable Chemistry & Engineering, 2019, 7(7): 7421-7429. DOI: 10.1021/acssuschemeng. 9b00991.
[128] SHIN S S, YANG W S, YEOM E J, LEE S J, JEON N J, JOO Y C, PARK I J, NOH J H, SEOK S Ⅱ. Tailoring of electron-collecting oxide nanoparticulate layer for flexible perovskite solar cells [J]. The Journal of Physical Chemistry Letters, 2016, 7(10): 1845-1851. DOI: 10.1021/acs.jpclett. 6b00295.
[129] SHIN S S, YEOM E J, YANG W S, HUR S, KIM M G, IM J, SEO J, NOH J H, SEOK S II. Colloidally prepared la-doped BaSnO3 electrodes for efficient, photostable perovskite solar cells [J]. Science, 2017, 356(6334): 167-171. DOI: 10.1126/ science.aam6620.
[130] SHIN S S, SUK J H, KANG B J, YIN W P, LEE S J, NOH J H, AHN T K, ROTERMUND F, CHO I S, SEOK S II. Energy-level engineering of the electron transporting layer for improving open-circuit voltage in dye and perovskite-based solar cells [J]. Energy & Environmental Science, 2019. DOI: 10.1039/C8EE03672A.
[131] GUO Heng, CHEN Hai-yuan, ZHANG Hai-yan, HUANG Xu, YANG Jian, WANG Bo-jun, LI Yu-lan, WANG Li-ping, NIU Xiao-bin, WANG Zhi-ming. Low-temperature processed yttrium-doped SrSnO3 perovskite electron transport layer for planar heterojunction perovskite solar cells with high efficiency [J]. Nano Energy, 2019, 59: 1-9. DOI: 10.1016/j.nanoen.2019.01.059.
[132] LIU Jiang, GAO Cheng, LUO Li-zhu, YE Qin-yan, HE Xu-lin, OUYANG Liang-qi, GUO Xiao-wei, ZHUANG Da-ming, LIAO Cheng, MEI Jun, LAU W. Low-temperature, solution processed metal sulfide as an electron transport layer for efficient planar perovskite solar cells [J]. Journal of Materials Chemistry A, 2015, 3(22): 11750-11755. DOI: 10.1039/C5TA01200G.
[133] DONG Jia, WU Ji-huai, JIA Jin-biao, FAN Le-qing, LIN Yu, LIN Jian-ming, HUANG Miao-liang. Efficient perovskite solar cells employing a simply-processed CdS electron transport layer [J]. Journal of Materials Chemistry C, 2017, 5(38): 10023-10028. DOI: 10.1039/C7TC03343E.
[134] XU Zhe, WU Ji-huai, YANG Yu-qian, LAN Zhang, LIN Jian-ming. High-efficiency planar hybrid perovskite solar cells using indium sulfide as electron transport layer [J]. ACS Applied Energy Materials, 2018, 1(8): 4050-4056. DOI: 10.1021/acsaem.8b00726.
[135] YIN Guan-nan, ZHAO Huan, FENG Jiang-shan, SUN Jie, YAN Jun-qing, LIU Zhi-ke, LIN Sheng-huang, LIU Sheng-zhong (Frank). Low-temperature and facile solution-processed two-dimensional TiS2 as an effective electron transport layer for UV-stable planar perovskite solar cells [J]. Journal of Materials Chemistry A, 2018, 6(19): 9132-9138. DOI: 10.1039/C8TA01143E.
[136] HUANG Peng, YUAN Li-gang, ZHANG Kai-cheng, CHEN Qiao-yun, ZHOU Yi, SONG Bo, LI Yong-fang. Room- temperature and aqueous solution-processed two- dimensional TiS2 as an electron transport layer for highly efficient and stable planar n–i–p perovskite solar cells [J]. ACS Applied Materials & Interfaces, 2018, 10(17): 14796-14802. DOI: 10.1021/acsami.8b03225.
[137] ZHAO Xiao-juan, LIU Shuang-shuang, ZHANG Hai-tao, CHANG Sheng-yung, HUANG Wen-chao, ZHU Bo-wen, SHEN Yan, SHEN Cai, WANG De-yu, YANG Yang, WANG Ming-kui. 20% efficient perovskite solar cells with 2D electron transporting layer [J]. Advanced Functional Materials, 2019, 29(4): 1805168. DOI: 10.1002/adfm. 201805168.
[138] KIM H S, LEE C R, IM J H, LEE K B, MOEHL T, MARCHIORO A, MOON S J, HUMPHRY-BAKER R, YUM J H, MOSER J E, GRATZEL M, PARK N G. Lead iodide perovskite sensitized all-solid-state submicron thin film mesoscopic solar cell with efficiency exceeding 9% [J]. Scientific Reports, 2012, 2: 591. DOI: 10.1038/srep00591.
[139] JIANG Qi, CHU Ze-ma, WANG Peng-yang, YANG Xiao-lei, LIU Heng, WANG Ye, YIN Zhi-gang, WU Jin-liang, ZHANG Xing-wang, YOU Jing-bi. Planar-structure perovskite solar cells with efficiency beyond 21% [J]. Advanced Materials, 2017, 29(46): 1703852. DOI: 10.1002/adma.201703852.
[140] HEO J H, IM S H, NOH J H, MANDAL T N, LIM C S, CHANG J A, LEE Y H, KIM H J, SARKAR A, NAZEERUDDIN M K, GRATZEL M, SEOK S II. Efficient inorganic–organic hybrid heterojunction solar cells containing perovskite compound and polymeric hole conductors [J]. Nature Photonics, 2013, 7(6): 486-491. DOI: 10.1038/nphoton.2013.80.
[141] WANG Zhi-ping, LIN Qian-qian, WENGER B, CHRISTOFORO M G, LIN Y H, KLUG M T, JOHNSTON M B, HERZ L M, SNAITH H J. High irradiance performance of metal halide perovskites for concentrator photovoltaics [J]. Nature Energy, 2018, 3(10): 855-861. DOI: 10.1038/s41560-018-0220-2.
[142] SHI Hui, LIU Cong-cong, JIANG Qing-lin, XU Jing-kun. Effective approaches to improve the electrical conductivity of PEDOT:PSS: A review [J]. Advanced Electronic Materials, 2015, 1(4): 1500017. DOI: 10.1002/aelm.201500017.
[143] HWANG J, AMY F, KAHN A. Spectroscopic study on sputtered PEDOT·PSS: Role of surface PSS layer [J]. Organic Electronics, 2006, 7(5): 387-396. DOI: 10.1016/ j.orgel.2006.04.005.
[144] LI Gang, ZHU Rui, YANG Yang. Polymer solar cells [J]. Nature Photonics, 2012, 6(3): 153-161. DOI: 10.1038/ nphoton.2012.11.
[145] YANG Jun-liang, YAN Dong-hang, JONES T S. Molecular template growth and its applications in organic electronics and optoelectronics [J]. Chemical Reviews, 2015, 115(11): 5570-5603. DOI: 10.1021/acs.chemrev.5b00142.
[146] YANG Jun-liang, YAN Dong-hang. Weak epitaxy growth of organic semiconductor thin films [J]. Chemical Society Reviews, 2009, 38(9): 2634. DOI: 10.1039/b815723p.
[147] WANG Chun-hua, LI Yuan, ZHANG Chu-jun, SHI Li-yang, TONG Si-chao, GUO Bin, ZHANG Jian, HE Jun, GAO Yong-li, SU Chao-hao, YANG Jun-liang. Enhancing the performance of planar heterojunction perovskite solar cells using stable semiquinone and amine radical modified hole transport layer [J]. Journal of Power Sources, 2018, 390: 134-141. DOI: 10.1016/j.jpowsour.2018.04.049.
[148] HUANG Ke-qing, WANG Chun-hua, ZHANG Chu-jun, TONG Si-chao, LI Heng-yue, LIU Biao, GAO Ya-xin, DONG Ya-nan, GAO Yong-li, PENG Yong-yi, YANG Jun-liang. Efficient and stable planar heterojunction perovskite solar cells fabricated under ambient conditions with high humidity [J]. Organic Electronics, 2018, 55: 140-145. DOI: 10.1016/j.orgel.2018.01.029.
[149] HUANG Ju, WANG Chun-hua, LIU Zi-ye, QIU Xue-qing, YANG Jun-liang, CHANG Jing-jing. Simultaneouly enhanced durability and performance by employing dopamine copolymerized PEDOT with high work function and water-proofness for inverted perovskite solar cells [J]. Journal of Materials Chemistry C, 2018, 6(9): 2311-2318. DOI: 10.1039/C7TC05276F.
[150] WANG Chun-hua, ZHANG Chu-jun, TONG Si-chao, SHEN Jian-qiang, WANG Can, LI You-zhen, XIAO Si, HE Jun, ZHANG Jian, GAO Yong-li, YANG Jun-liang. Air-induced high-quality CH3NH3PbI3 thin film for efficient planar heterojunction perovskite solar cells [J]. The Journal of Physical Chemistry C, 2017, 121(12): 6575-6580. DOI: 10.1021/acs.jpcc.7b00981.
[151] WANG Chun-hua, ZHANG Chu-jun, HUANG Yu-lan, TONG Si-chao, WU Han, ZHANG Jian, GAO Yong-li, YANG Jun-liang. Degradation behavior of planar heterojunction CH3NH3PbI3 perovskite solar cells [J]. Synthetic Metals, 2017, 227: 43-51. DOI: 10.1016/ j.synthmet.2017.02.022.
[152] WU Run-sheng, YANG Jun-liang, XIONG Jian, LIU Peng, ZHOU Cong-hua, HUANG Han, GAO Yong-li, YANG Bing-chu. Efficient electron-blocking layer-free planar heterojunction perovskite solar cells with a high open-circuit voltage [J]. Organic Electronics, 2015, 26: 265-272. DOI: 10.1016/j.orgel.2015.07.057.
[153] WU Run-sheng, YANG Bing-chu, ZHANG Chu-jun, HUANG Yu-lan, CUI Yan-xia, LIU Peng, ZHOU Cong-hua, HAO Yu-ying, GAO Yong-li, YANG Jun-liang. Prominent efficiency enhancement in perovskite solar cells employing silica-coated gold nanorods [J]. The Journal of Physical Chemistry C, 2016, 120(13): 6996-7004. DOI: 10.1021/acs. jpcc.6b00309
[154] XIONG Jian, YANG Bing-chu, WU Run-sheng, CAO Cheng-hao, HUANG Yu-lan, LIU Cheng-bin, HU Zhi-kun, HUANG Han, GAO Yong-li, YANG Jun-liang. Efficient and non-hysteresis CH3NH3PbI3/PCBM planar heterojunction solar cells [J]. Organic Electronics, 2015, 24: 106-112. DOI: 10.1016/j.orgel.2015.05.028.
[155] LIU Yao, BAG M, RENNA L A, PAGE Z A, KIM P, EMRICK T, VENKATARAMAN D, RUSSELL T P. Understanding interface engineering for high-performance fullerene/perovskite planar heterojunction solar cells [J]. Advanced Energy Materials, 2016, 6(2): 1501606. DOI: 10.1002/aenm.201501606.
[156] XIONG Jian, YANG Jun-liang, YANG Bing-chu, ZHOU Cong-hua, HU Xu, XIE Hai-peng, HUANG Han, GAO Yong-li. Efficient and stable inverted polymer solar cells using TiO2 nanoparticles and analysized by Mott-Schottky capacitance [J]. Organic Electronics, 2014, 15(8): 1745-1752. DOI: 10.1016/j.orgel.2014.04.041.
[157] XIONG Jian, YANG Bing-chu, YUAN Jun, FAN Ling, HU Xu, XIE Hai-peng, LYU Lu, CUI Rui-li, ZOU Ying-ping, ZHOU Cong-hua, NIU Dong-mei, GAO Yong-li, YANG Jun-liang. Efficient organic photovoltaics using solution- processed, annealing-free TiO2 nanocrystalline particles as an interface modification layer [J]. Organic Electronics, 2015, 17: 253-261. DOI: 10.1016/j.orgel.2014.12.023.
[158] XIONG Jian, YANG Bing-chu, ZHOU Cong-hua, YANG Jun-liang, DUAN Hai-chao, HUANG Wen-long, ZHANG Xiang, XIA Xing-da, ZHANG Lei, HUANG Han, GAO Yong-li. Enhanced efficiency and stability of polymer solar cells with TiO2 nanoparticles buffer layer [J]. Organic Electronics, 2014, 15(4): 835-843. DOI: 10.1016/j.orgel. 2014.01.024.
[159] XIONG Jian, YANG Bing-chu, CAO Cheng-hao, WU Run-sheng, HUANG Yu-lan, SUN Jia, ZHANG Jian, LIU Cheng-bin, TAO Shao-hua, GAO Yong-li, YANG Jun-liang. Interface degradation of perovskite solar cells and its modification using an annealing-free TiO2 NPs layer [J]. Organic Electronics, 2016, 30: 30-35. DOI: 10.1016/ j.orgel.2015.12.010.
[160] CAO Cheng-hao, ZHANG Chu-jun, YANG Jun-liang, SUN Jia, PANG Shu-ping, WU Han, WU Run-sheng, GAO Yong-li, LIU Cheng-bin. Iodine and chlorine element evolution in CH3NH3PbI3–xClx thin films for highly efficient planar heterojunction perovskite solar cells [J]. Chemistry of Materials, 2016, 28(8): 2742-2749. DOI: 10.1021/acs. chemmater.6b00429.
[161] LIM K G, KIM H B, JEONG J, KIM H, KIM J Y, LEE T W. Boosting the power conversion efficiency of perovskite solar cells using self-organized polymeric hole extraction layers with high work function [J]. Advanced Materials, 2014, 26(37): 6461-6466. DOI: 10.1002/adma.201401775.
[162] ZUO Chuan-tian, DING Li-ming. Modified PEDOT layer makes a 1.52 V Voc for perovskite/PCBM solar cells [J]. Advanced Energy Materials, 2017, 7(2): 1601193. DOI: 10.1002/aenm.201601193.
[163] LIU Dong-yang, LI Yong, YUAN Jian-yu, HONG Qiu-ming, SHI Guo-zheng, YUAN Da-xing, WEI Jian, HUANG Chen-chao, TANG Jian-xin, FUNG M K. Improved performance of inverted planar perovskite solar cells with F4-TCNQ doped PEDOT:PSS hole transport layers [J]. Journal of Materials Chemistry A, 2017, 5(12): 5701-5708. DOI: 10.1039/C6TA10212C.
[164] JIANG Kui, WU Fei, ZHANG Guang-ye, Y.CHOW P C, MA Chao, LI Shu-fang, SINGWONG K, ZHU Lin-na, YAN He. Inverted planar perovskite solar cells based on CsI-doped PEDOT:PSS with efficiency beyond 20% and small energy loss [J]. Journal of Materials Chemistry A, 2019, 7(38): 21662-21667. DOI: 10.1039/C9TA08995K.
[165] XIA Yi-jie, SUN Kuan, CHANG Jing-jing, OUYANG Jian-yong. Effects of organic inorganic hybrid perovskite materials on the electronic properties and morphology of poly(3,4-ethylenedioxythiophene):poly(styrenesulfonate) and the photovoltaic performance of planar perovskite solar cells [J]. Journal of Materials Chemistry A, 2015, 3(31): 15897- 15904. DOI: 10.1039/C5TA03456F.
[166] MALINKIEWICZ O, YELLA A, LEE Y HIN M K, BOLINK H J. Perovskite solar cells employing organic charge-transport layers [J]. Nature Photonics, 2014, 8(2): 128-132. DOI: 10.1038/nphoton.2013.341.
[167] HOCKER J, KIERMASCH D, RIEDER P, TVINGSTEDT K, BAUMANN A, DYAKONOV V. Efficient solution processed CH3NH3PbI3 perovskite solar cells with polytpd hole transport layer [J]. Zeitschrift für Naturforschung A, 2019, 74(8): 665-672. DOI: 10.1515/zna-2019-0127.
[168] INTANIWET A, KEDDIE J L, SHKUNOV M, SELLIN P J. High charge-carrier mobilities in blends of poly(triarylamine) and TIPS-pentacene leading to better performing X-ray sensors [J]. Organic Electronics, 2011, 12(11): 1903-1908. DOI: 10.1016/j.orgel.2011.08.003.
[169] LEEM D S, WOBKENBERG P, HUANG Jing-song, ANTHOPOULOS T D, BRADLEY D D C, DEMELLO J C. Micron-scale patterning of high conductivity poly(3,4- ethylendioxythiophene):poly(styrenesulfonate) for organic field-effect transistors [J]. Organic Electronics, 2010, 11(7): 1307-1312. DOI: 10.1016/j.orgel.2010.04.002.
[170] ZHENG Xiao-peng, DENG Ye-hao, CHEN Bo, WEI Hao-tong, XIAO Xun, FANG Yan-jun, LIN Yu-ze, YU Zhen-hua, LIU Ye, WANG Qi, HUANG Jin-song. Dual functions of crystallization control and defect passivation enabled by sulfonic zwitterions for stable and efficient perovskite solar cells [J]. Advanced Materials, 2018, 30(52): 1803428. DOI: 10.1002/adma.201803428.
[171] WANG Qi, BI Cheng, HUANG Jin-song. Doped hole transport layer for efficiency enhancement in planar heterojunction organolead trihalide perovskite solar cells [J]. Nano Energy, 2015, 15: 275-280. DOI: 10.1016/j.nanoen. 2015.04.029.
[172] SHAO Yu-chuan, YUAN Yong-bo, HUANG Jin-song. Correlation of energy disorder and open-circuit voltage in hybrid perovskite solar cells [J]. Nature Energy, 2016, 1(1): 15001. DOI: 10.1038/nenergy.2015.1.
[173] YOU Jing-bi, MENG Lei, SONG T B, GUO T F, YANG Yang (Michael), CHANG W H, HONG Zi-ruo, CHEN Hua-jun, ZHOU Huan-ping, CHEN Qi, LIU Yong-sheng, DE MARCO N, YANG Yang. Improved air stability of perovskite solar cells via solution-processed metal oxide transport layers [J]. Nature Nanotechnology, 2016, 11(1): 75-81. DOI: 10.1038/nnano.2015.230.
[174] ZUO Chuan-tian, DING Li-ming. Solution-processed Cu2O and CuO as hole transport materials for efficient perovskite solar cells [J]. Small, 2015, 11(41): 5528-5532. DOI: 10.1002/smll.201501330
[175] JENG J Yn, CHEN K C, CHIANG T Y, LIN P Y, TSAI T D, CHANG Y C, GUO T F, CHEN P, WEN T C, HSU Y J. Nickel oxide electrode interlayer in CH3NH3PbI3 perovskite/PCBM planar-heterojunction hybrid solar cells [J]. Advanced Materials, 2014, 26(24): 4107-4113. DOI: 10.1002/adma.201306217.
[176] CHEN Wei, WU Yong-zhen, YUE You-feng, LIU Jian, ZHANG Wen-jun, YANG Xu-dong, CHEN Han, BI En-bing, ASHRAFUL I, GRATZEL M, HAN Li-yuan. Efficient and stable large-area perovskite solar cells with inorganic charge extraction layers [J]. Science, 2015, 350(6263): 944-948. DOI: 10.1126/science.aad1015
[177] CHEN Wei, ZHOU Ye-cheng, WANG Lin-jing, WU Ying-hui, TU Bao, YU Bin-bin, LIU Fang-zhou, TAM H W, WANG Gan, DJURISIC A B, HUANG Li, HE Zhu-bing. Molecule-doped nickel oxide: verified charge transfer and planar inverted mixed cation perovskite solar cell [J]. Advanced Materials, 2018, 30(20): 1800515. DOI: 10.1002/adma.201800515.
[178] CHOWDHURY T H, AKHTARUZZAMAN M, KAYESH M Emrul, KANEKO R, NODA T, LEE J J, ISLAM A. Low temperature processed inverted planar perovskite solar cells by r-GO/CuSCN hole-transport bilayer with improved stability [J]. Solar Energy, 2018, 171: 652-657. DOI: 10.1016/j.solener.2018.07.022.
[179] YE Sen-yun, SUN Wei-hai, LI Yun-long, YAN Wei-bo, PENG Hai-tao, BIAN Zu-qiang, LIU Zhi-wei, HUANG Chun-hui. CuSCN-based inverted planar perovskite solar cell with an average pce of 15.6% [J]. Nano Letters, 2015, 15(6): 3723-3728. DOI: 10.1021/acs.nanolett.5b00116.
[180] YANG Dao-bin, SANO T, YAGUCHI Y, SUN H, SASABE H, KIDO J. Achieving 20% efficiency for low-temperature- processed inverted perovskite solar cells [J]. Advanced Functional Materials, 2019, 29(12): 1807556. DOI: 10.1002/adfm.201807556.
[181] CHIANG C H, TSENG Z L, WU C G. Planar heterojunction perovskite/PC71BM solar cells with enhanced open-circuit voltage via a (2/1)-step spin-coating process [J]. Journal of Materials Chemistry A, 2014, 2(38): 15897-15903. DOI: 10.1039/C4TA03674C.
[182] CHEN Chun-chao, HONG Zi-rou, LI Gang, CHEN Qi, ZHOU Huan-ping, YANG Yang. One-step, low-temperature deposited perovskite solar cell utilizing small molecule additive [J]. Journal of Photonics for Energy, 2015, 5(1): 057405. DOI: 10.1117/1.JPE.5.057405.
[183] GUERRERO A, YOU Jing-bi, ARANDA C, KANG Y S, GARCIA-BELMONTE G, ZHOU Huan-ping, BISQUERT J, YANG Yang. Interfacial degradation of planar lead halide perovskite solar cells [J]. ACS Nano, 2016, 10(1): 218-224. DOI: 10.1021/acsnano.5b03687.
[184] XIA Fei, WU Qi-liang, ZHOU Peng-cheng, LI Yi, CHEN Xiang, LIU Qing, ZHU Jun, DAI Song-yuan, LU Ya-lin, YANG Shang-feng. Efficiency enhancement of inverted structure perovskite solar cells via oleamide doping of PCBM electron transport layer [J]. ACS Applied Materials & Interfaces, 2015, 7(24): 13659-13665. DOI: 10.1021/acsami. 5b03525.
[185] KUANG Chao-yang, TANG Gang, JIU Tong-gang, YANG Hui, LIU Hui-biao, LI Bai-ru, LUO Wei-ning, LI Xiao-dong, ZHANG Wen-jun, LU Fu-shen, FANG Jun-feng, LI Yu-liang. Highly efficient electron transport obtained by doping PCBM with graphdiyne in planar-heterojunction perovskite solar cells [J]. Nano Letters, 2015, 15(4): 2756-2762. DOI: 10.1021/acs.nanolett.5b00787.
[186] CHEN Ke, HU Qin, LIU Tang-hao, ZHAO Li-chen, LUO De-ying, WU Jiang, ZHANG Yi-fei, ZHANG Wei, LIU Feng, RUSSELL T P, ZHU Rui, GONG Qi-huang. Charge-carrier balance for highly efficient inverted planar heterojunction perovskite solar cells [J]. Advanced Materials, 2016, 28(48): 10718-10724. DOI: 10.1002/adma.201604048.
[187] XIE Cheng-yi, ZHOU Cong-hua, BIN YANG, SHEN Liang, KE Li-li, DING Li-ming, YUAN Yong-bo. Silicon phthalocyanine passivation for fullerene-free perovskite solar cells with efficient electron extraction [J]. Applied Physics Express, 2019, 12(6): 064006. DOI: 10.7567/1882-0786/ ab1fb6
[188] CHEN Cheng, LI Hong-ping, DING Xing-dong, CHENG Ming, LI He-nan, XU Li, QIAO Fen, LI Hua-ming, SUN Li-cheng. Molecular engineering of triphenylamine-based non-fullerene electron-transport materials for efficient rigid and flexible perovskite solar cells [J]. ACS Applied Materials & Interfaces, 2018, 10(45): 38970-38977. DOI: 10.1021/ acsami.8b15130
[189] BAILIE C D, CHRISTOFORO M G, MAILOA J P, BOWRING A R, UNGER E L, NGUYEN W H, BURSCHKA J, PELLET N, LEE J Z, GRATZEL M, NOUFI R, BUONASSISI T, SALLEO A, MCGEHEE M D. Semi-transparent perovskite solar cells for tandems with silicon and CIGS [J]. Energy & Environmental Science, 2015, 8(3): 956-963. DOI: 10.1039/C4EE03322A.
[190] AHN J, HWANG H, JEONG S, MOON J. Metal-nanowire- electrode-based perovskite solar cells: Challenging issues and new opportunities [J]. Advanced Energy Materials, 2017, 7(15): 1602751. DOI: 10.1002/aenm.201602751.
[191] CHEN Hai-ning, YANG Shi-he. Carbon-based perovskite solar cells without hole transport materials: The front runner to the market? [J]. Advanced Materials, 2017, 29(24): 1603994. DOI: 10.1002/adma.201603994.
[192] DAI Xue-zeng, ZHANG Ye, SHEN He-ping, LUO Qiang, ZHAO Xing-yue, LI Jian-bao, LIN Hong. Working from both sides: Composite metallic semitransparent top electrode for high performance perovskite solar cells [J]. ACS Applied Materials & Interfaces, 2016, 8(7): 4523-4531. DOI: 10.1021/acsami.5b10830.
[193] LEE M, KO Y, MIN B K, JUN Y. Silver nanowire top electrodes in flexible perovskite solar cells using titanium metal as substrate [J]. ChemSusChem, 2016, 9(1): 31-35. DOI: 10.1002/cssc.201501332.
[194] ZHANG Jian-hua, LI Fu-shan, YANG Kai-yu, VEERAMALAI C P, GUO Tai-liang. Low temperature processed planar heterojunction perovskite solar cells employing silver nanowires as top electrode [J]. Applied Surface Science, 2016, 369: 308-313. DOI: 10.1016/j.apsusc. 2016.02.104
[195] HAN Kang, XIE Meng-lan, ZHANG Lian-ping, YAN Ling-peng, WEI Jun-feng, JI Guo-qi, LUO Qun, LIN Jian, HAO Yu-ying, MA Chang-qi. Fully solution processed semi-transparent perovskite solar cells with spray-coated silver nanowires/ZnO composite top electrode [J]. Solar Energy Materials and Solar Cells, 2018, 185: 399-405. DOI: 10.1016/j.solmat.2018.05.048.
[196] XIE Meng-lan, LU Hui, ZHANG Lian-ping, WANG Jie, LUO Qun, LIN Jian, BA Li-xiang, LIU Hong, SHEN Wen-zhong, SHI Li-yi, MA Chang-qi. Fully solution- processed semi-transparent perovskite solar cells with ink-jet printed silver nanowires top electrode [J]. Solar RRL, 2018, 2(2): 1700184. DOI: 10.1002/solr.201700184.
[197] GRANCINI G, ROLDAN-CARMONA C, ZIMMERMANN I, MOSCONI E, LEE X, MARTINEAU D, NARBEY S, OSWALD F, DE ANGELIS F, GRAETZEL M, NAZEERUDDIN M K. One-year stable perovskite solar cells by 2D/3D interface engineering [J]. Nature Communications, 2017, 8(1): 1-8. DOI: 10.1038/ ncomms15684.
[198] WANG Qi-fei, ZHANG Wen-hao, ZHANG Zhi-hui, LIU Shuang, WU Jia-wen, GUAN Yan-jun, MEI An-yi, RONG Yao-guang, HU Yue, HAN Hong-wei. Crystallization control of ternary-cation perovskite absorber in triple-mesoscopic layer for efficient solar cells [J]. Advanced Energy Materials, 2020, 10(5): 1903092. DOI: 10.1002/aenm.201903092.
[199] WEI Zhan-hua, CHEN Hai-ning, YAN Ke-you, YANG Shi-he. Inkjet printing and instant chemical transformation of a CH3NH3PbI3/nanocarbon electrode and interface for planar perovskite solar cells [J]. Angewandte Chemie International Edition, 2014, 53(48): 13239-13243. DOI: 10.1002/anie. 201408638.
[200] ZHANG Fu-guo, YANG Xi-chuan, WANG Hao-xin, CHENG Ming, ZHAO Jiang-hua, SUN Li-cheng. Structure engineering of hole–conductor free perovskite-based solar cells with low-temperature-processed commercial carbon paste as cathode [J]. ACS Applied Materials & Interfaces, 2014, 6(18): 16140-16146. DOI: 10.1021/am504175x.
[201] ZHOU Hua-wei, SHI Yan-tao, DONG Qing-shun, ZHANG Hong, XING Yu-jin, WANG Kai, DU Yi, MA Ting-li. Hole-conductor-free, metal-electrode-free TiO2/CH3NH3PbI3 heterojunction solar cells based on a low-temperature carbon electrode [J]. The Journal of Physical Chemistry Letters, 2014, 5(18): 3241-3246. DOI: 10.1021/jz5017069.
[202] ZHANG Chen-xi, LUO Yu-dan, CHEN Xiao-hong, CHEN Yi-wei, SUN Zhuo, HUANG Su-mei. Effective improvement of the photovoltaic performance of carbon-based perovskite solar cells by additional solvents [J]. Nano-Micro Letters, 2016, 8(4): 347-357. DOI: 10.1021/jz5017069.
[203] JIN Yi, CHUMANOV G. Solution-processed planar perovskite solar cell without a hole transport layer [J]. ACS Applied Materials & Interfaces, 2015, 7(22): 12015-12021. DOI: 10.1021/acsami.5b02124.
[204] DENG Ye-hao, BRACKLE C H V, DAI Xue-zeng, ZHAO Jing-jing, CHEN Bo, HUANG Jin-song. Tailoring solvent coordination for high-speed, room-temperature blading of perovskite photovoltaic films [J]. Science Advances, 2019, 5(12): 7537. DOI: 10.1126/sciadv.aax7537.
(Edited by HE Yun-bin)
中文导读
溶液法制备钙钛矿太阳电池
摘要:钙钛矿太阳电池已经成为光伏应用中最有前途的候选者之一。它具有低成本、低温溶液涂布或印刷工艺制备及兼容大规模卷对卷制造工艺等优点,展现出巨大的商业化前景。在这篇文章中,我们重点综述了介孔和平面结构钙钛矿太阳电池器件中溶液法沉积电荷传输层、钙钛矿吸收层及顶电极层。此外,还重点概括了可大规模溶液法制备钙钛矿薄膜的工艺方法、钙钛矿薄膜结晶性增强及表面缺陷钝化等,进而提高钙钛矿太阳电池性能。
关键词:钙钛矿太阳电池;介孔结构;平面结构;溶液法制备;大规模制备
CHANG Jian-hui, LIU Kun and LIN Si-yuan contributed equally to this work.
Foundation item: Projects(51673214, 51673218, 61774170) supported by the National Natural Science Foundation of China; Project(2017YFA0206600) supported by the National Key Research and Development Program of China
Received date: 2020-01-14; Accepted date: 2020-02-28
Corresponding author: YANG Jun-liang, PhD, Professor; Tel: +86-731-88660256; E-mail: junliang.yang@csu.edu.cn; ORCID: 0000- 0002-5553-0186; YUAN Yong-bo, PhD, Professor; E-mail: yuanyb@csu.edu.cn; ORCID: 0000-0002-4606- 4611; ZHOU Cong-hua, PhD, Professor; E-mail: chzhou@csu.edu.cn; ORCID: 0000-0002-7565-9016
Abstract: Perovskite solar cells (PSCs) have emerged as one of the most promising candidates for photovoltaic applications. Low-cost, low-temperature solution processes including coating and printing techniques makes PSCs promising for the greatly potential commercialization due to the scalability and compatibility with large-scale, roll-to-roll manufacturing processes. In this review, we focus on the solution deposition of charge transport layers and perovskite absorption layer in both mesoporous and planar structural PSC devices. Furthermore, the most recent design strategies via solution deposition are presented as well, which have been explored to enlarge the active area, enhance the crystallization and passivate the defects, leading to the performance improvement of PSC devices.
- Solution-processed perovskite solar cells
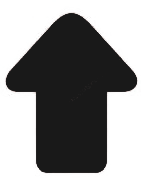