- Abstract:
- 1 Introduction▲
- 2 Experimental▲
- 3 Results and discussion▲
- 4 Conclusions▲
- References
- Figure
- Fig.1 FT-IR spectrum of niobium ethoxide
- Fig.2 Raman spectrum of niobium ethoxide
- Fig.3 1H NMR spectrum of niobium ethoxide sample (A and B represent serial number of H atom)
- Fig.4 TG-DTG plots for benzoic acid (a) and niobium ethoxide (b)
- Fig.5 Vapour pressure-temperature curve of niobium ethoxide
- Fig.6 Plot of ln p versus 1/T for niobium ethoxide
J. Cent. South Univ. Technol. (2011) 18: 73-77
DOI: 10.1007/s11771-011-0661-2
Electrochemical synthesis, characterization and thermal properties of niobium ethoxide
CAI Ya-nan(蔡亚楠)1, YANG Sheng-hai(杨声海)2, JIN Sheng-ming(金胜明)1,
YANG Hai-ping(杨海平)2, HOU Guo-feng(侯国锋)2, XIA Jiao-yun(夏姣云)2
1. School of Minerals Processing and Bioengineering, Central South University, Changsha 410083, China;
2. School of Metallurgical Science and Engineering, Central South University, Changsha 410083, China
? Central South University Press and Springer-Verlag Berlin Heidelberg 2011
Abstract:
Niobium(V) ethoxide (Nb(OEt)5) was synthesized by electrochemical reaction of ethanol with niobium plate as the sacrificial anode, stainless steel as the cathode and tetraethylammonium chloride (TEAC) as the conductive additive. The condensates were isolated by vacuum distillation under 5 kPa. The product was characterized by Fourier transform infrared (FT-IR) spectra, Raman spectra and nuclear magnetic resonance (NMR) spectra. The results indicate that the product is niobium ethoxide. Thermal properties of niobium ethoxide were analysed by TG/DTG. Vapour pressure was calculated from the Langmuir equation and the enthalpy of vaporization was calculated from the vapour pressure-temperature data using the Clausius–Clapeyron equation. The concentrations of impurity metallic elements in the sample were detected by ICP-MS. It is shown that the purity can reach 99.997%. The volatility and purity of the niobium ethoxide ensure that it could be a good precursor for chemical vapor deposition and atomic layer deposition of niobium oxide layers.
Key words:
electrochemical synthesis; niobium ethoxide; characterization; thermal analysis;
1 Introduction
Niobium ethoxide can be utilized for the deposition of niobium oxide layers by chemical vapor deposition (CVD), and therefore is valuable starting compound for producing extremely robust components which have applications, for example, in the electronics and optical industry [1-5]. Such metal oxide layers can also be produced from the niobium ethoxide via hydrolysis using a sol-gel method [6-7]. The very high dielectric constant enables the use of niobium oxide layers in dynamic random access read/write memories (DRAMs) [8]. LiNbO3 thin films prepared from Nb(OEt)5 are important in developing new optical devices and largely-integrated electronic circuits because of their non-linear optical and ferroelectric properties [9-10]. Also, in the electric lamp industry, niobium oxide which can be deposited by CVD plays a role, for example, in the production of incandescent bulbs.
Niobium pentachloride is usually employed as the raw material in the present technique for the synthesis of niobium alkoxide which is the product of the reaction between niobium pentachloride and appropriate alcohol in diluted solvent of benzene or toluene [11]. As metal halide reacts with alcohol only at partial replacement, it is necessary to add some reagents like ammonia or pyridine to promote the completion of the reaction. In order to remove the trace halide dissolved in alcohol and niobium alkoxides, alkali metals are added to form metal halides. In that case, traditional preparation method has some disadvantages, such as restricted requirements on equipments, bad work condition, low recovery ratio and toxic diluted solvent pollution.
Significant progress has been made in the electrochemical synthesis of metallorganic complexes in the last several decades. In our earlier works, we have prepared several tantalum alkoxides by electrochemical method [12-13]. In contrast to the conventional methods, the electrochemical synthetic process is more productive and inexpensive with regards to apparatus and raw materials. Furthermore, it is more friendly to the environment. Niobium ethoxide was obtained for the first time by SHREIDER et al [14] with the electrochemical method involving electrolysis of an ethanol solution of triethylamine hydrobromide with a platinum cathode and a niobium anode. The electrochemical reaction process and dissolution mechanism were investigated by BEREZKIN et al [15]. However, characterization and thermal property analysis of niobium ethoxide have not been undertaken so far.
In the present work, Nb(OEt)5 was directly prepared with niobium plate of industrial grade as a sacrificial anode in absolute alcohol. It was characterized by several methods including FT-IR, Raman spectra, NMR, thermal analysis and ICP-MS.
2 Experimental
2.1 Materials and equipments
Anhydrous ethanol (AR grade) was purchased from Tianjin Hengxing Chemical Preparation Co., Ltd, China. Tetraethylammonium chloride (TEAC) was purchased from Jintan Huadong Chemical Research Institute of Jiangsu Province, China. All reagents were used without further purification.
The electrolytic cell is made of polypropylene and its dimensions are 15.1 cm(length)×7 cm(width)×24 cm (height). A stainless steel plate was used as cathode, and its working area was 19.0 cm×12.0 cm. An niobium plate (1.63 kg), purchased from Zhuzhou Cement Carbide Group Limited Corporation, China, was employed as the anode, and its working area was 18.0 cm×13.0 cm. The niobium plate was made from niobium powders of metallurgical grade with self-resistance sintering, electron bombardment and rolling treatment. The chemical composition of the niobium plate is listed in Table 1. The DC electrical source (WYJ 15A-60V) was supplied by Shanghai Wenkai Power Equipment Co., Ltd, China.
Table 1 Chemical composition of niobium anode (mass fraction, %)
2.2 Synthesis and isolation
Electrochemical synthesis was performed in a diaphragmless plastic cell, equipped with a condenser. Solution of TEAC (0.04 mol/L) in the ethanol was used as electrolyte. The two electrodes were polished with a series of emery papers, degreased in acetone, rinsed in deionized water and ethanol. The process was performed under direct current with refluxing of the electrolyte and hydrogen evolution at the cathode. Voltage of 60 V and process duration of 24-48 h were applied depending on the yield wanted. The current efficiency was calculated to be above 96%.
Firstly, distillation was performed at ambient pressure to separate redundant ethanol from mixed solutions of ethanol and niobium ethoxide at temperature above the boiling point of ethanol. Then, the distillation temperature was raised to 150 °C gradually, and a little amount of ester was removed. Finally, the crude niobium ethoxide solution was distilled at a pressure of 5 kPa and an oil bath temperature of 210-220 °C. The condensate was preserved in a dry nitrogen-sealed glass bottle to keep it from moisture.
2.3 Characterization
FT-IR spectrum was measured with a Nicolet Avatar 360 IR spectrometer operating in the region of 4 000-400 cm-1. Raman spectrum was recorded using a Laboram-010 laser Raman spectrometer with 632.8 nm He-Ne laser. The sampling time was 1 s and the samples were sealed in a glass tube in inert gas ambient. The 1H-NMR spectrum was recorded with an Inova-400 (Varian) nuclear magnetic resonance spectroscope, and chloroform-d was adopted as the dilute reagent. Thermogravimetric measurements were carried out in nitrogen atmosphere using a SDT Q600 V8.0 Build 95 thermoanalytical equipment (flux rate 200 cm3/min, heating rate 10 K/min, temperature interval 25-800 °C, sample mass 21.8 mg, and surface area of the TG crucible 0.227 cm2). The impurity metal contents of the hydrolyzate Nb2O5 were determined by ICP-MS Agilent 7500A.
3 Results and discussion
3.1 Structure characterization
Fig.1 shows the FT-IR spectrum of niobium ethoxide. The peaks between 2 969 cm-1 and 2 861 cm-1 are due to δ(C—H) stretch vibration of alkoxy groups. Peaks at around 2 969 cm-1 and 2 861 cm-1 are ascribed to asymmetric and symmetric stretch vibrations, respectively. The sharp bands at around 1 470 and 1 375 cm-1 correspond to the δ(C—H) bend vibrations. Peak at 1 470 cm-1 is attributed to scissoring vibration of methylene. Peak at around 1 375 cm-1 is attributed to
Fig.1 FT-IR spectrum of niobium ethoxide
symmetric bending vibration of methyl groups. Bands at 1 200-1 000 cm-1 correspond to the δ(C—O) vibrations of alkoxy groups bound to Nb. The broad envelope of band at 571 cm-1 is due to the δ(Nb—O) stretching modes occurring along with bending and torsional modes of the ligands [16].
The Raman spectrum of niobium ethoxide is shown in Fig.2. The peak at 591 cm-1 could be assigned to δ(Nb—O) vibrations, and the bands around 1 077 cm-1 are attributed to the Nb(—O—C) stretching vibrations [17]. The peaks between 2 800 and 2 970 cm-1 are ascribed to the C—H vibrations of the alkyl groups. They are in good agreement with the results of FT-IR spectrum.
Fig.2 Raman spectrum of niobium ethoxide
Nuclear magnetic resonance studies on niobium ethoxide are very useful in understanding and assigning the structure of the compound. Fig.3 shows the 1H NMR spectrum of Nb(OEt)5 in CDCl3 with respect to tetramethylsilane. Solvent peaks are observed at 7.26× 10-6. Peak assignments in the plot are as follows: the signals at 4.4×10-6 are assigned to HA. The major peak at 1.2×10-6 is due to HB, namely the H atom of the terminal methyl. The proportion of spectral integral area is 1.19:
Fig.3 1H NMR spectrum of niobium ethoxide sample (A and B represent serial number of H atom)
1.80≈2:3, conforming the stoichiometry of the compound Nb(OCH2CH3)5. The spin quantum number of niobium is 9/2, and the peak of —CH2— splits to multiplets according to the coupling splitting rules. The H—H coupling effect between methyl and methylene also leads to splitting of the two peaks [16].
3.2 Thermal properties analysis and vapour pressure estimation
Volatility is of prime consideration in assessing the feasibility of a metallorganic compound as a precursor in chemical vapor deposition and it determines the conditions required to transport material from its source container to the deposition zone [5, 18].
In this work, we use the Langmuir equation to calculate the vapour pressure and the vaporization enthalpy of niobium ethoxide. The principle of using thermogravimetry to estimate the vapour pressure is based on the Langmuir equation [19]:
(1)
where dm/dt(a) is the rate of mass loss per unit area (kg·s-1·m-2), p the vapour pressure (Pa), M the relative molecular mass of the vapour of the evaporating compound (kg·mol-1), R the gas constant (J·K-1·mol-1), T the absolute temperature (K), and α the vaporization coefficient. In vacuum, α is assumed to be 1, but in a flowing gas atmosphere as commonly found in TG experiments α is assumed to be a significantly different value. Rearranging the Langmuir equation gives
p=kv (2)
where and
The usual procedure using TG is to determine the rate of mass loss over the temperature range of interest using a compound known to be thermally stable. Knowing the vapour pressure of the standard compound at different temperatures, and obtaining dm/dt from TG/DTG plots, the value of k is determined. Benzoic acid has been suggested as a suitable material for this role [20-21]. Then, it is used as the calibrating material for the determination of k in the modified Langmuir equation.
The enthalpy of vaporization is calculated from the vapour pressure-temperature data using the Clausius– Clapeyron equation:
(3)
Hence, a plot of ln p versus 1/T gives a straight line, the slope of which is -?H/R. Thus, the enthalpy of vaporization may be calculated.
The TG/DTG plots for benzoic acid and Nb(OEt)5 sample are shown in Fig.4. The TG curve of Nb(OEt)5 displays a single mass loss in the temperature range 413- 523 K. On the DTG plot, the rate of mass loss shows a rapid increase after reaching 453 K. The rate of mass loss continues to increase as the sample evaporates, reaching the maximum at the DTG peak temperature of 509 K. With an empty sample crucible and a total mass loss of 95% upon completion of the TG experiment, it is concluded that niobium ethoxide has almost completely evaporated.
Fig.4 TG-DTG plots for benzoic acid (a) and niobium ethoxide (b)
From the plot of benzoic acid, the values of dm/dt at different temperatures are obtained, and divided by the surface area of the TG crucible to get the mass loss rate per unit surface area. k is calculated to be 1.547×105 J0.5·K-0.5·mol-0.5 as required by Eq.(1). Using this value of k, the vapour pressure-temperature curve for niobium ethoxide is calculated, as shown in Fig.5. From the vapour pressure-temperature plots for the precursor, it is observed that Nb(OEt)5 has large variation of vapour pressure over a wide range of temperature. The vapour pressure reaches 21.5 kPa when the temperature rises to 500 K. This indicates that the complex Nb(OEt)5 could be good precursor for CVD, because it would allow great control over the precursor flux during the deposition process.
Using the vapour pressure-temperature data, plot of ln p versus 1/T is obtained as straight line for niobium ethoxide, from which, using the Clausius–Clapeyron equation, the slope gives -?H/R, and hence the vaporization enthalpy ?H. The plot of ln p versus 1/T for niobium ethoxide is shown in Fig.6. The linear fitting to the data, the slope of which gives the enthalpy of sublimation, is also shown in Fig.6. The linear nature of this curve allows the vaporization enthalpy to be calculated from the slope, and the value is found to be 75.1 kJ/mol for niobium ethoxide. The vapour pressure- temperature equation for niobium ethoxide can be expressed as
ln p=28.1-9 027.2/T
Fig.5 Vapour pressure-temperature curve of niobium ethoxide
Fig.6 Plot of ln p versus 1/T for niobium ethoxide
3.3 Purity characterization
Niobium ethoxide is an useful starting material for preparing oxides usable for dielectrics or optical materials. When such an oxide is used as an electronic material for dielectrics, it is required to reduce the quantity of impurities such as transition metals typified by iron, alkali metals e.g. sodium, alkaline earth metals e.g. calcium, as well as uranium and thorium as far as possible. These impurity elements cause various disorders such as the reduction in the durability of electronic devices, and the increase in the leakage current and software errors [22]. The concentrations of impurity elements in the sample are shown in Table 2. The content of all other impurity elements except for Ta is about 10×10-6 (mass fraction). Therefore, the purity of niobium ethoxide purified by reduced pressure distillation can be up to 99.997% and can meet the demand of metal- organic chemical vapor deposition (MOCVD). The content of Ta is relatively high whereas the element tantalum is not more harmful to the quality than other elements.
Table 2 Impurity contents in niobium ethoxide (mass fraction, 10-6)
4 Conclusions
1) It is demonstrated that Nb(OEt)5 can be prepared by electrochemical synthesis. In comparison with the traditional chemical methods of synthesis of niobium ethoxide, electrochemistry method appears to be rather simple and productive.
2) The structure of the product was characterized by FT-IR, Raman and NMR spectra. The results consistently demonstrate that the sample is niobium ethoxide.
3) The vapour pressure-temperature equation for niobium ethoxide can be expressed as ln p=28.1- 9 027.2/T. The vaporization enthalpy of niobium ethoxide is found to be 75.1 kJ/mol. The result confirms that Nb(OEt)5 is suitable for chemical vapor deposition. Purity characterization indicates that the purity of niobium ethoxide purified by reduced pressure distillation can be up to 99.997%.
References
[1] MASSE J P, SZYMANOWSKI H, ZABEIDA O, AMASSIAN A, KLEMBERG-SAPIEHA J E, MARTINU L. Stability and effect of annealing on the optical properties of plasma-deposited Ta2O5 and Nb2O5 films [J]. Thin Solid Films, 2006, 515(4): 1674-1682.
[2] TANAKA A, MIYASHITA K, TASHIRO T, KIMURA M, SUKEGAWA T. Preparation of lithium niobate films by metalorganic chemical vapor deposition with a lithium alkoxide source [J]. Journal of Crystal Growth, 1995, 148(3): 324-326.
[3] MARUYAMA T, KANAGAWA T. Electrochromic properties of niobium oxide thin films prepared by chemical vapor deposition [J]. Journal of the Electrochemical Society, 1994, 141(10): 2868-2871.
[4] WERNBERG A A, GYSLING H J. Chemical vapor deposition of niobium and tantalum oxide films: US, 5271957 [P]. 1993-12-21.
[5] BRADLEY D C. Metal alkoxides as precursors for electronic and ceramic materials [J]. Chemical Reviews, 2002, 89(6): 1317-1322.
[6] WOOD B D, MOCANU V, GATES B D. Solution-phase synthesis of crystalline lithium niobate nanostructures [J]. Advanced Materials, 2008, 20(23): 4552-4556.
[7] MACEK M, OREL B. Electrochromism of sol-gel derived niobium oxide films [J]. Solar Energy Materials and Solar Cells, 1998, 54(1): 121-130.
[8] PIGNOLET A, RAO G M, KRUPANIDHI S B. Rapid thermal processed thin films of niobium pentoxide (Nb2O5) deposited by reactive magnetron sputtering [J]. Thin Solid Films, 1995, 261(1/2): 18-24.
[9] AKIYAMA Y, SHITANAKA K, MURAKAMI H, SHIN Y S, YOSHIDA M, IMAISHI N. Epitaxial growth of lithium niobate film using metalorganic chemical vapor deposition [J]. Thin Solid Films, 2007, 515(12): 4975-4979.
[10] TONG Hai-xia, CHEN Qi-yuan, HU Hui-ping, YIN Zhou-lan, LI Jie, ZHOU Jian-liang. Preparation, characterization and photocatalytic behavior of WO3-TiO2/Nb2O5 catalysts [J]. Journal of Central South University of Technology, 2007, 14(6): 788-792.
[11] ZHANG Jian-cheng, ZHOU Hai-li, CHEN Ya-li, SHEN Yue, ZHANG Yi-hua. Analysis of synthetical conditions, hydrolytic process and their characteristics for niobium alkoxides [J]. Journal of Shanghai University, 1998, 4(1): 38-44. (in Chinese)
[12] YANG Sheng-hai, CHEN Yong-ming, YANG Hai-ping, LIU Yin-yuan, TANG Mo-tang, QIU Guan-zhou. Preparation of high-purity tantalum ethoxide by vacuum distillation [J]. Transactions of Nonferrous Metals Society of China, 2008, 18(1): 196-201.
[13] YANG Sheng-hai, CAI Ya-nan, YANG Hai-ping, Jin Sheng-ming. Electrochemical synthesis and characterization of tantalum alkoxides [J]. Transactions of Nonferrous Metals Society of China, 2009, 19(6): 1504-1508.
[14] SHREIDER V A, TUREVSKAYA E P, KOSLOVA N I, TUROVA N Y. Direct electrochemical synthesis of metal alkoxides [J]. Inorganica Chimica Acta, 1981, 53: L73-L76.
[15] BEREZKIN M Y, CHERNYKH I N, POLYAKOV E G, TOMILOV A P. Electrochemical synthesis of niobium(V) ethylate [J]. Applied Electrochemistry and Corrosion Protection of Metals, 2006, 79(5): 752-756.
[16] SILVERSTEIN R M, WEBSTER F X, KIEMLE D. Spectrometric identification of organic compounds [M] New York: John Wiley & Sons, 2005: 82-101.
[17] DOLLISH F R, FATELEY W G, BENTLEY F F. Characteristic Raman frequencies of organic compounds [M]. New York: Wiley, 1974: 218.
[18] RUSHWORTH S, DAVIES H, KINGSLEY A, LEESE T, ODEDRA R. Volatility and vapourisation characterisation of new precursors [J]. Microelectronics Reliability, 2007, 47: 718-721.
[19] LANGMUIR I. The vapor pressure of metallic tungsten [J]. Physical Review, 1913, 2: 329-342.
[20] KUNTE G V, SHIVASHANKAR S A, UMARJI A M. Thermogravimetric evaluation of the suitability of precursors for MOCVD [J]. Measurement Science and Technology, 2008, 19(2): 1-7.
[21] WRIGHT S F, DOLLIMOREL D, DUNN J G, ALEXANDER K. Determination of the vapor pressure curves of adipic acid and triethanolamine using thermogravimetric analysis [J]. Thermochimica Acta, 2004, 421: 25-30.
[22] HOCHIDO Y, KADOKURA H, TANABE S, KOBAYASHI R. Process for purifying niobium alkoxides and tantalum alkoxides: US, 5919963 [P]. 1999-07-06.
Foundation item: Project(2007AA03Z425) supported by the National Hi-tech Research and Development Program of China; Project(50404011) supported by the National Natural Science Foundation of China
Received date: 2009-12-04; Accepted date: 2010-03-01
Corresponding author: YANG Sheng-hai, Associate Professor, PhD; Tel: +86-731-88830470; E-mail: 75894838@163.com
- Electrochemical synthesis, characterization andthermal properties of niobium ethoxide
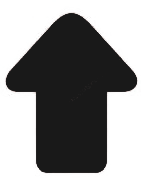