- Abstract:
- 1 Introduction▲
- 2 Experimental setup▲
- 3 Data processing me...▲
- 4 Results and discus...▲
- 5 Conclusions▲
- References
- Figure
- Figure 1 PLIF system and combustion system
- Figure 2 Schematic diagram of flame height, flame kernel cone height and reaction area
- Figure 3 OH distribution of waste oil biodiesel (a), diesel (b) and ethanol (c) diffusion flame (Unit: mL/min)
- Figure 4 Relationship between flame kernel cone height and fuel flow rate
- Figure 5 Relationship between flame height and fuel flow rate
- Figure 6 Relationship between average OH signal intensity of three kinds of fuels and fuel flow rate
- Figure 7 OH distribution of combustion flame of biodiesel and ethanol blended fuels
- Figure 8 Change of flame kernel cone height under different ethanol addition ratio
- Figure 9 Change of flame height under different ethanol addition ratio
- Figure 10 Change of average OH signal intensity under different ethanol addition ratio
J. Cent. South Univ. (2018) 25: 1043-1051
DOI: https://doi.org/10.1007/s11771-018-3804-x
Effect of ethanol addition on flame characteristics of waste oil biodiesel
GAO Jin(高进)1, LI Fa-she(李法社)1, 2, ZHANG Xiao-hui(张小辉)1, 2,WANG Hua(王华)2, FENG Zong-hong(冯宗红)1, SHEN Yi-cheng(申逸骋)1
1. Faculty of Metallurgical and Energy Engineering, Kunming University of Science and Technology, Kunming 650093, China;
2. State Key Laboratory of Complex Nonferrous Metal Resources Clean Utilization,Kunming University of Science and Technology, Kunming 650093, China
Central South University Press and Springer-Verlag GmbH Germany, part of Springer Nature 2018
Abstract:
Biodiesel is a kind of clean and renewable energy. The effect of ethanol addition on the flame characteristics of waste oil biodiesel is studied by using OH-PLIF technique from the perspective of OH radical evolution. Ethanol addition leads to the appearance of diffusion flame reaction interface ahead of schedule and shortens the diffusion flame height. The experimental results show a linear correlation between the flame height and the fuel flow rate for a given fuel and oxidant. The same conclusion is drawn from the theoretical analysis of the approximate model. In addition, ethanol addition makes the average OH signal intensity of flame at different fuel flow rate tend to be consistent and the fuel flow rate enlarge where the flame field shows the strongest oxidation performance. Average OH signal intensity begins to weaken at larger fuel flow rate, which indicates that fuel flow rate of fuels blended with ethanol can change in larger range and does not significantly affect the uniformity of combustion.
Key words:
waste oil biodiesel; ethanol; diffusion flame; OH radical; PLIF;
Cite this article as:
GAO Jin, LI Fa-she, ZHANG Xiao-hui, WANG Hua, FENG Zong-hong, SHEN Yi-cheng. Effect of ethanol addition on flame characteristics of waste oil biodiesel [J]. Journal of Central South University, 2018, 25(5): 1043–1051.
DOI:https://dx.doi.org/https://doi.org/10.1007/s11771-018-3804-x1 Introduction
With the further development of economy, the world's energy consumption is also increasing. Traditional fossil energy reserves are limited. We need to seek an alternative energy in engine in order to solve the problem of energy shortage [1]. Biodiesel is a kind of clean and renewable energy, and it has great potential in the field of alternative to traditional fossil fuels [2]. The research of biodiesel combustion includes combustion mechanism, combustion stability and emission characteristics and so on.
OH radical is an important intermediate product in the combustion process, which can identify the combustion reaction area more accurately and which is commonly exploited to study the flame structure and propagation velocity, the turbulent flame characteristics, the heat release rate, the local extinguished and OH radical concentration distribution [3–7]. Planar laser induced fluorescence (PLIF) is a kind of laser diagnostic technique, which is commonly used to study the evolution of OH radical. PLIF can be used to study the flame characteristics and combustion mechanism [8, 9]. Hydrocarbon fuels are easy to produce OH radical when burning [10], and biodiesel fuels contain hydrocarbons, so it is desirable to study the combustion of biodiesel through PLIF technique. SANG et al [11] and WON et al [12] proposed that there existed a close relationship between free radicals concentration, especially OH radical concentration, and extinguish, a linear relationship having been found between extinction stretch rate and the maximum OH concentration. FRANZELLI et al [13] pointed out that the OH radical can oxidize soot in its vicinity, namely a larger amount of soot implies a smaller amount of OH. ALVISO et al [8] studied the biodiesel combustion mechanism through PLIF technique. In addition to studying the flame structure under normal pressure, the OH radical can also be used to study the flame structure in high pressure environment [10].
The basic research about the combustion of biodiesel fuels from the perspective of free radical evolution is rare. In recent years, the typical research is to study the combustion mechanism of biodiesel or biodiesel alternative fuels by counter flow diffusion flame [8]. In the research of engine, a large number of scholars have studied the combustion of biodiesel, including combustion of neat biodiesel, biodiesel and diesel blended fuels and biodiesel and ethanol blended fuels [14–16]. Because of the change of the waste oil biodiesel composition with the change of the area, the waste oil biodiesel in the combustion use may show the phenomenon of combustion instability, which leads to incomplete combustion and low efficiency combustion. In order to deal with this problem, it is an attempt to add ethanol to the waste oil biodiesel to improve the combustion process. This paper uses PLIF technique to study OH radical distribution of diffusion flame of the waste oil biodiesel, diesel and biodiesel and ethanol blended fuels and analyzes the effect of ethanol addition on the combustion flame characteristics of waste cooking oil biodiesel. The research results can provide reference for reducing the generation of soot and improving the uniformity of combustion.
2 Experimental setup
The OH-PLIF flame detection system employed in this work is shown in Figure 1(a). It mainly consists of a Nd: YAG laser (Model: LAB-170-10H) with power of 200 mJ per pulse and a pulse time of 10 ns, a Sirah dye laser (Model: CBST-G-30-EG) producing output wavelength of 283.36 nm used to excite OH radical, and a CCD camera (CCD Type: Imager LX2M 1600×1200 7.4 μm; Intensifier Type: 25 mm V7670U-70- P43) equipped with a UV lens and an OH filter. The working environment temperature of the OH-PLIF system is 22 °C, and the indoor humidity is 60%RH.
Under laboratory conditions, there are many kinds of the liquid flame generators, such as wick combustion [17, 18], gas atomization combustion [19, 20] and electric atomization combustion [21]. The combustion device used in this experiment is the wick-fed combustion device, mainly comprising a syringe pump (LongerPump, Model: LSP01-2A), ceramic wick holder and wick (see Figure 1(b)). The wick is essentially a glass fiber that can withstand temperatures of 1000 °C and has the advantages of good oil guiding performance. The wick used can timely transport fuels to wick surface, even at a maximum fuel flow rate (0.18 mL/min). Injection pump can provide fuels continuously and stably. The ceramic wick holder can keep the combustion stable.
Figure 1 PLIF system and combustion system
3 Data processing method
Because of the transient nature of the flame, a time-averaged acquisition method is widely used in their study [22, 23]. However, transient flame images are adopted for the wick-fed diffusion flame, because of its flame stability. In this work, 500 transient pictures are captured at each fuel flow rate, and then the 500 pictures are averaged, and the background is removed. After background subtraction, some image intensity values become negative, mainly due to mismatch between the acquired background and the actual background information. To correct this error, all negative values are set to 0. Since the processed images contain non-integer values, we applied the “float to word” function in the DaVis 8.2 software to convert all values into integers. This experiment uses transient images, when the flame remains stable. In Figure 2, red lines represents equal strength curve (counts=2). Flame kernel cone height, flame height and reaction zone are determined based on those red lines. In Figure 2, (a1, b1) represents pixel position of the flame tip, (a2, b2) pixel position of flame kernel cone tip, and (a0, b0) pixel position of the wick nozzle. Before the experiment, the ratio of pixels to the actual size was calibrated, and the calibration factor is X=0.1013 mm/pixel. Therefore, flame kernel cone height H=|b2–b0|·X, and flame height Hf=|b1–b0|·X. The average OH signal intensity of the flame is defined as the average value of the OH signal intensity in the flame reaction region. The closed area of the red line in Figure 2 represents the reaction zone. According to the definition, the average OH signal intensity where
denotes sum of the intensity in the reaction zone and n denotes sum pixel in reaction region.
Figure 2 Schematic diagram of flame height, flame kernel cone height and reaction area
4 Results and discussions
4.1 OH distribution characteristics of different fuel combustion flames
OH is one of the free radicals produced in the combustion process of hydrocarbon fuels. The existence of OH denotes the combustion reaction [3, 4]. OH can be used to determine the combustion reaction zone and the basic structure of the combustion flame. The formation of OH is related to the combustion process and fuel species. Figure 3 shows the OH distribution of waste oil biodiesel, 0# diesel and ethanol diffusion flame. In Figure 3, the OH distribution of waste oil biodiesel combustion flame is basically similar to that of diesel combustion flame. The OH distribution of ethanol combustion flame is significantly different from that of the waste oil biodiesel and diesel combustion flame. The ethanol combustion shows a larger flame core area, where the concentration of OH radical is very low, with a relatively uniform OH intensity distribution.
The basic structure of diffusion flame is influenced by fuel types. The combustion process of fuels in a wick-fed burner includes the following basic processes. (a) The wick capillary force drives fuels to flow upward; (b) Flame heat evaporates fuels on wick surface; (c) Fuel vapor moves to flame surface and decomposes into smaller hydrocarbons; (d) Oxygen from ambient environment moves to flame by convection and diffusion; (e) Combustion of combustible substances and oxygen occurs in the flame area. Flame kernel cone height and flame height are determined from Figure 3. Figure 4 shows the relationship between flame kernel cone height and fuel flow rate. Figure 5 shows the relationship between flame height and fuel flow rate. Flame kernel cone height and flame height of three fuels increase with the increase of fuel flow rate. Flame kernel core height of ethanol combustion flame is significantly higher than that of the waste oil biodiesel and diesel combustion flame. Waste oil biodiesel and diesel flame have OH radical signal near the wick nozzle, probably because a small portion of the fuel vapor is decomposed to produce OH radical. However, the situation of ethanol combustion flame is different. The ethanol molecule structure is simple, and the ethanol vapor diffusion rate is larger, entering the area far from the nozzle for combustion. In Figure 5, minor differences of combustion flame height of three kinds of fuels are observed in smaller fuel flow rate range, but when fuel flow rate is greater than 0.14 mL/min, flame height of ethanol is significantly higher than that of the other two kinds of fuels.
Figure 3 OH distribution of waste oil biodiesel (a), diesel (b) and ethanol (c) diffusion flame (Unit: mL/min)
Figure 4 Relationship between flame kernel cone height and fuel flow rate
Figure 5 Relationship between flame height and fuel flow rate
Combustion flame height is an important characteristic parameter of diffusion flame. The diffusion flame height can also be analyzed theoretically. Considering an approximate model shown in Figure 1(b), a non-dimensional analysis of the species and energy equations is developed below. Define non-dimensional quantities as
(1)
where ws is the fuel mass fraction when fuel and oxidant is mixed with stoichiometric. If the Cs indicates the fuel concentration of a stoichiometric mixture,
(2)
Species mass fraction:
(3)
where vfis fuel consumption rate per unit volume; D is diffusivity.
Energy equation:
(4)
where Q is the heat of combustion per mole of fuel burned. With Eqs. (1) and (2), Eq. (4) can be simplified to
(5)
Assuming that τconv=Hf/v represents the convective time of the flame, represents diffusion time of oxygen to the center of the axis and
represents reaction time, Eq. (5) can be simplified to
(6)
With Eqs. (1) and (2), Eq. (3) is reduced to
(7)
where τconv/τchem denotes ratio between convection time and reaction time. When the reaction rate is fast, the value becomes infinite, and the reaction characteristic is controlled by the transportation process. τconv/τdiff denotes ratio between convection time and diffusion time. At the flame tip, these two times are approximately equal, so
(8)
where qv is fuel volume flow rate. For a given fuel and an oxidizing agent, i.e. D is constant, the flame height and fuel volume flow rate are linearly dependent, and this relationship can be seen in Figure 5. In addition, the flame height also depends on fuel and oxidant types [24, 25]. There are many kinds of biodiesels, and the height of combustion flame is also influenced by the type of fuel [26].
The average OH signal intensity is a measure of the average OH concentration in the two- dimensional flame plane. OH radical has a very strong ability to get electrons, i.e. oxidation ability. The greater the average OH concentration in the combustion field, the stronger the oxidation ability of the combustion field, which is beneficial to the oxidation of soot and polycyclic aromatic hydrocarbons [11, 12]. Figure 6 shows the relationship between the average OH signal intensity of the three kinds of fuels and fuel flow rate. The average OH signal intensity in ethanol and waste oil biodiesel combustion flame is first increased and then decreased, with the increase of fuel flow rate; the average OH signal intensity of the diesel flame is increased with the increase of the fuel flow rate in the test range. At smaller fuel flow rate, the combustion thermal condition is poor, and the combustion process is not stable, so the flames of the three kinds of fuels show weaker OH signal intensity. With the increase of fuel flow rate in smaller range, the average OH signal strength increases, but with further increase, the production of soot and other components caused by incomplete combustion starts to increase, which leads to the consumption of OH radical and further reduction of average OH signal strength. In Figure 6, the average OH signal intensity of diesel flame has not shown a decreasing trend in the test fuel flow rate.
Figure 6 Relationship between average OH signal intensity of three kinds of fuels and fuel flow rate
4.2 Effect of ethanol addition on OH distribution of combustion flame of waste oil biodiesel
The component of waste oil is complex, so the composition of the waste oil biodiesel varies greatly. In order to optimize the combustion process of waste oil biodiesel, the ethanol can be added to the waste oil biodiesel. Adding ethanol may affect the combustion process and the combustion flame characteristics. Figure 7 shows OH distribution of combustion flame of biodiesel and ethanol blended fuels.
Figure 7 OH distribution of combustion flame of biodiesel and ethanol blended fuels
In Figure 7, three important parameters are extracted, i.e. flame kernel cone height, flame height and average OH signal intensity. The changes of the three parameters are shown in Figures 8, 9 and 10. From Figure 8, it can be concluded that when fuel flow is 0.1 mL/min, the flame kernel cone height of neat biodiesel and mixed fuels is approximately 0 mm. When fuel flow rate changes in a smaller range, there is enough air around the fire to spread into the flame, so the flame core area is very small. When fuel flow rate changes in the range larger than 0.14 mL/min, the change rate of flame kernel core height of mixed fuels is greater than that of the neat biodiesel.
Figure 8 Change of flame kernel cone height under different ethanol addition ratio
Figure 9 Change of flame height under different ethanol addition ratio
Figure 10 Change of average OH signal intensity under different ethanol addition ratio
Figure 9 shows the change of flame height of waste oil biodiesel blended with ethanol. Figure 9 shows that when the fuel flow is less than 0.177 mL/min, adding ethanol in the waste oil biodiesel makes the flame height decrease, and flame height increase with increasing ethanol addition ratio. Just as discussed in Section 4.1, waste oil biodiesel combustion flame kernel cone height is lower than ethanol combustion flame kernel cone height, which means that near the wick nozzle occurs reaction in the biodiesel flame. In the combustion process of mixed fuels, ethanol enters the reaction zone near the wick nozzle created by waste oil biodiesel combustion, which makes the reaction of ethanol occur ahead of schedule, and the combustion of ethanol in turn provide good thermodynamic conditions for combustion reaction. Ethanol addition makes the combustion of mixed fuels occur ahead of schedule, causing shortened flame height. In addition, because ethanol is oxygenated fuel, ethanol can improve the oxygen- deficient environment near the wick nozzle, which is conducive to the rapid combustion, reducing the post combustion phenomenon, resulting in a high degree of flame height reduction. However, with the increase of ethanol in the fuel, the diffusivity of the mixed fuels increases and the flame height begins to increase.
The stronger the average OH signal intensity of in the flame, the greater the concentration of OH radical in the combustion field. At a certain position in the flame field, the greater the concentration of the OH radical in the position, the greater the oxidation ability in the position contributed by the OH radical. Figure 10 shows the change of the average OH signal intensity in the mixed fuels combustion flame. As shown in Figure 6, the average OH signal intensity in waste oil biodiesel and ethanol combustion flame increases at first and then decreases with the increase of fuel flow rate, and a similar trend can be observed in Figure 10. It is shown from Figure 10 that when adding ethanol to waste oil biodiesel, the maximum value of the average OH signal intensity in the combustion flame can be reduced, while the minimum value of average OH signal intensity has little change, which means that the difference of the average OH signal intensity in flame at each fuel flow rate is reduced, indicating that the difference of the average OH concentration in flame at different fuel flow rate is also reduced, or that the oxidation ability contributed by the OH radical in the flame field has little difference. Here the analysis shows that in the test fuel flow rate range, adding ethanol can make the flame field enhance the ability to maintain the oxidation performance. For neat waste oil biodiesel combustion, with the change of fuel flow rate, the oxidation performance in combustion field contributed by the OH radical changes greatly, and the flame is not uniform, easy to cause incomplete combustion. From Figure 10 it can be found that for the waste oil biodiesel combustion flame, when the fuel flow is about 0.12 mL/min, the average OH signal strength reaches a maximum value, whereas for mixed fuels, when the fuel flow is about 0.14 mL/min, the average OH signal intensity reaches the maximum, indicating that the adding ethanol in waste oil biodiesel increases fuel flow rate where the strongest oxidation performance appears. Average OH signal intensity begins to weaken at larger fuel flow rate, which indicates that fuel flow rate of fuels blended with ethanol can change in a larger range and does not significantly affect the uniformity of combustion.
5 Conclusions
The influence of ethanol addition on the combustion flame characteristics of the waste oil biodiesel is studied by using PLIF technique and the intermediate product OH radical as the medium. The following conclusions are drawn. Ethanol combustion flame kernel cone height and the kernel cone area are bigger than the waste oil biodiesel and the diesel combustion flame, and OH radical distribution in ethanol combustion flame is also relatively uniform. The experimental results show a linear correlation between the flame height and the fuel flow rate for a given fuel and oxidant. The same conclusion is drawn from the theoretical analysis of the approximate model. The average OH signal intensity in ethanol and waste oil biodiesel combustion flame is first increased and then decreased, with the increase of fuel flow rate; the average OH signal intensity of the diesel flame is increased with the increase of the fuel flow rate in the test range. Ethanol addition makes the average OH signal intensity of flame at different fuel flow rate tend to be consistent. Average OH signal intensity begins to weaken at larger fuel flow rate, which indicates that fuel flow rate of fuels blended with ethanol can change in larger range and does not significantly affect the uniformity of combustion.
References
[1] GHAJAR M, KAKAEE A H, MASHADI B. Semi-empirical modeling of volumetric efficiency in engines equipped with variable valve timing system [J]. Journal of Central South University, 2016, 23(12): 3132–3142. DOI: 10.1007/ s11771-016-3379-3.
[2] HABIBULLAH M, MASJUKI H H, KALAM M A. Potential of biodiesel as a renewable energy source in Bangladesh [J]. Renewable and Sustainable Energy Reviews, 2015, 50: 819–834. DOI: 10.1016/j.rser.2015.04.149.
[3] KHALIL A E E, GUPTA A K. Hydroxyl radical distribution in distributed reaction combustion condition [J]. Fuel, 2014, 122(15): 28–35. DOI: 10.1016/j.fuel.2014.01.010.
[4] YAMAMOTO K, ISII S, OHNISHI M. Local flame structure and turbulent burning velocity by joint PLIF imaging [J]. Proceedings of the Combustion Institute, 2011, 33(1): 1285–1292. DOI: 10.1016/j.proci.2010.06.087.
[5] LI Z S, LI B, SUN Z W. Turbulence and combustion interaction: High resolution local flame front structure visualization using simultaneous single-shot PLIF imaging of CH, OH, and CH2 O in a piloted premixed jet flame [J]. Combustion and Flame, 2010, 157(6): 1087–1096. DOI: 10.1016/j.combustflame.2010.02.017.
[6] YANG Li, WANG Zhi-hua, ZHU Yan-qun, LI Zhong-shan. Premixed jet flame characteristics of syngas using OH planar laser induced fluorescence [J]. Chinese Science Bulletin, 2011, 56(26): 2862–2868. DOI: 10.1007/s11434-011-4630-9.
[7] YUAN R, KARIUKI J, DOWLUT A. Reaction zone visualisation in swirling spray n-heptane flames [J]. Proceedings of the Combustion Institute, 2014, 35(2): 1649–1656. DOI: 10.1016/j.proci.2014.06.012.
[8] ALVISO D, ROLON J C, SCOUFLAIRE P. Experimental and numerical studies of biodiesel combustion mechanisms using a laminar counterflow spray premixed flame [J]. Fuel, 2015, 153: 154–165. DOI: 10.1016/j.fuel.2015.02.079.
[9] ZHANG M, WANG J, XIE Y. Measurement on instantaneous flame front structure of turbulent premixed CH4/H2/air flames [J]. Experimental Thermal and Fluid Science, 2014, 52(1): 288–296. DOI: 10.1016/j.expthermflusci.2013.10.002.
[10] MATYNIA A, IDIR M, MOLET J. Absolute OH concentration profiles measurements in high pressure counterflow flames by coupling LIF, PLIF, and absorption techniques [J]. Applied Physics B: Lasers and Optics, 2012, 108(2): 393–405. DOI: 10.1007/s00340-012-4959-z.
[11] SANG H W, DOOLEY S, DRYER F L. Kinetic effects of aromatic molecular structures on diffusion flame extinction [J]. Proceedings of the Combustion Institute, 2011, 33(1): 1163–1170. DOI: 10.1016/j.proci.2010.05.082.
[12] WON S H, SUN W, JU Y. Kinetic effects of toluene blending on the extinction limit of n-decane diffusion flames [J]. Combustion and Flame, 2010, 157(3): 411–420. DOI: 10.1016/j.combustflame.2009.11.016.
[13] FRANZELLI B, SCOUFLAIRE P, CANDEL S. Time- resolved spatial patterns and interactions of soot, PAH and OH in a turbulent diffusion flame [J]. Proceedings of the Combustion Institute, 2014, 35(2): 1921–1929. DOI: 10.1016/j.proci.2014.06.123.
[14] PARASARA M U, ADROJA F N. An experimental investigation of palm oil blend with diesel fuel on engine performance and emission of a diesel engine: A review [J]. Journal of Thermal Engineering and Application, 2015, 2(2): 1–8.
[15] QI D H, CHEN H, GENG L M. Performance and combustion characteristics of biodiesel–diesel–methanol blend fuelled engine [J]. Applied Energy, 2010, 87(5): 1679–1686. DOI: 10.1016/j.apenergy.2009.10.016.
[16] MUTHURAMAN S. The performance of four stroke surface ignition ceramic heater C.I. engine using ethanol-diesel blend [J]. International Journal of Energy and Power Engineering, 2014, 3(2): 38–45. DOI: 10.11648/j.ijepe. 20140302.11.
[17] MERCHAN-MERCHAN W, WARE H O T. Study of carbon and carbon–metal particulates in a canola methyl ester air-flame [J]. Combustion and Flame, 2015, 162(1): 216–225. DOI: 10.1016/j.combustflame.2014.07.007.
[18] BOTERO M L, MOSBACH S, AKROYD J. Sooting tendency of surrogates for the aromatic fractions of diesel and gasoline in a wick-fed diffusion flame [J]. Fuel, 2015, 153(3): 31–39. DOI: 10.1016/j.fuel.2015.02.108.
[19] KHOSOUSI A, LIU F, DWORKIN S B. Experimental and numerical study of soot formation in laminar coflow diffusion flames of gasoline/ethanol blends [J]. Combustion and Flame, 2015, 162(10): 3925–3933. DOI: 10.1016/ j.combustflame.2015.07.029.
[20] KONNOV A A, MEUWISSEN R J, GOEY L P H D. The temperature dependence of the laminar burning velocity of ethanol flames [J]. Proceedings of the Combustion Institute, 2011, 33(1): 1011–1019. DOI: 10.1016/j.proci.2010.06.143.
[21] TOSATTO L, MELLA F, LONG M B. A study of JP-8 surrogate coflow flame structure by combined use of laser diagnostics and numerical simulation [J]. Combustion and Flame, 2012, 159(10): 3027–3039. DOI: 10.1016/ j.combustflame.2012.05.001.
[22] ELBAZ A M, ROBERTS W L. Flame structure of methane inverse diffusion flame [J]. Experimental Thermal and Fluid Science, 2014, 56(5): 23–32. DOI: 0.1016/j.expthermflusci. 2013.11.011.
[23] LARTIGUE G, MEIER U, C. Experimental and numerical investigation of self-excited combustion oscillations in a scaled gas turbine combustor [J]. Applied Thermal Engineering, 2004, 24(11): 1583–1592. DOI: 10.1016/j.applthermaleng.2003.10.026.
[24] MCALLISTER S, CHEN J Y, FERNANDEZPELLO A C. Fundamentals of combustion processes [M]. New York: Springer, 2011. DOI: 10.1007/978-1-4419-7943-8_7.
[25] SUNDERLAND P B, QUINTIERE J G, TABAKA G A. Analysis and measurement of candle flame shapes [J]. Proceedings of the Combustion Institute, 2011, 33(2): 2489–2496. DOI: 10.1016/j.proci.2010.06.095.
[26] LI Fa-she, DU Wei, BAO Gui-rong. Improving research for low temperature fluidity of biodiesel [J]. Journal of Kunming University of Science and Technology, 2014, 39(5): 11–15. (in Chinese) DOI: 10.3969/j.issn.1007-855x.2014.05.003.
(Edited by YANG Hua)
中文导读
乙醇添加量对地沟油生物柴油燃烧火焰特性的影响
摘要:生物柴油是一种清洁可再生能源。本文应用OH-PLIF技术从OH自由基演化的角度研究了乙醇添加对地沟油生物柴油燃烧火焰特性的影响。添加乙醇会使扩散火焰反应界面前移,同时会降低扩散火焰高度。在燃料和氧化剂给定的情况下,试验和理论分析都表明燃油流率和火焰高度呈线性关系。添加乙醇会使火焰平均OH信号强度在火焰中分布更为均匀,且在燃油流率较大时火焰才会呈现出较强的氧化性。当燃油流率较大时,平均OH信号强度开始减弱,表明在地沟油生物柴油中添加乙醇时,燃油流率可在较大的燃油流率内变化,而不明显影响火焰均匀性。
关键词:地沟油生物柴油;扩散火焰;OH自由基;平面激光诱导荧光
Foundation item: Project(51766007) supported by the National Natural Science Foundation of China; Project(U1602272) supported by the NSFC-Yunnan Joint Fund Project; Project(2015FB128) supported by the Natural Science Fund Project in Yunnan Province, China; Project(CNMRCUTS1704) supported by the Research Fund from State Key Laboratory of Complex Nonferrous Metal Resources Clean Utilization, China
Received date: 2016-10-17; Accepted date: 2017-02-23
Corresponding author: LI Fa-she, PhD, Associate Professor; Tel: +86-15025131595; E-mail: asanli@foxmail.com; ORCID: 0000-0003- 1526-2524
Abstract: Biodiesel is a kind of clean and renewable energy. The effect of ethanol addition on the flame characteristics of waste oil biodiesel is studied by using OH-PLIF technique from the perspective of OH radical evolution. Ethanol addition leads to the appearance of diffusion flame reaction interface ahead of schedule and shortens the diffusion flame height. The experimental results show a linear correlation between the flame height and the fuel flow rate for a given fuel and oxidant. The same conclusion is drawn from the theoretical analysis of the approximate model. In addition, ethanol addition makes the average OH signal intensity of flame at different fuel flow rate tend to be consistent and the fuel flow rate enlarge where the flame field shows the strongest oxidation performance. Average OH signal intensity begins to weaken at larger fuel flow rate, which indicates that fuel flow rate of fuels blended with ethanol can change in larger range and does not significantly affect the uniformity of combustion.
- Effect of ethanol addition on flame characteristics of waste oil biodiesel
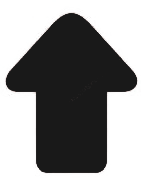