- Abstract:
- 1 Introduction▲
- 2 Experimental ▲
- 3 Results and discussion▲
- 4 Conclusions▲
- References
- Figure
- Fig.1 Loss tangent tan δ as function of temperature for PAN solution with 4% H2O at various angular frequencies (Above tgel, from top to bottom, ω=0.100, 0.398, 1.000, 3.980, 10.000 and 39.800 rad/s. tgel is the gel point.)
- Fig.2 Loss tangent tan δ as function of temperature for PAN solution with 6% H2O at various angular frequencies (Above tgel, from top to bottom, ω=0.100, 0.398, 1.000, 3.980, 10.000 and 39.800 rad/s. tgel is the gel point.)
J. Cent. South Univ. Technol. (2008) 15(s1): 122-125
DOI: 10.1007/s11771-008-329-8
Rheological behavior during thermotropic gelation of polyacrylonitrile concentrated solutions
MA Cai-xia(马彩霞)1, YAN Wei-xia(闫伟霞)2, ZHANG Li(张 莉)2, QIN Zong-yi(秦宗益)1, PAN Ding(潘 鼎)1
(1. State Key Laboratory for Chemical Fibers Modification and Polymer Materials, Donghua University, Shanghai 201620, China;
2. Research Centre for Analysis and Measurement, Donghua University, Shanghai 201620, China)
Abstract:
Dynamic viscoelastic properties of polyacrylonitrile(PAN)/DMSO/H2O solutions with different H2O contents were studied as a function of temperature. These PAN solutions gradually became gel with decreasing temperature. The sol-gel transition took place at a critical gel temperature, at which the scaling law of G′(ω)~G″(ω)∝ωn held, allowing an accurate determination of the critical gel temperature by means of the frequency independence of the loss tangent. The gel point of PAN solutions increases with increasing H2O content. The scaling exponent n (=0.86) at the gel point is confirmed to be universal for PAN gels, which is independent of temperature, suggesting the similarity of the fractal structure in the critical PAN gels.
Key words:
polyacrylonitrile; gel point; thermotropic gelation; rheological behavior;
1 Introduction
As well-known, a polymeric gel is a three -dimensional network forming from flexible chains through either chemical cross-linking or physical phase transformation. There is a difference between chemical and physical gels. Chemical gels are irreversible and their crosslinks have an infinite lifetime. In physical gels[1], many causes of crosslinking, for example, van der Waals forces, electrostatic attraction and hydrogen bonding. Crosshinking can be employed to bind polymer chains together to form a reversible gel network, in which the crosslinks have a finite lifetime. The gelation is such a phenomenon that a polymeric liquid dramatically becomes solidlike at a critical point of temperature, time, polymer concentration, etc. In other words, the sol-gel transition of polymers is a very important critical phenomena[2]. The well-used experimental and theoretical methods for description of the gelation are based on the scaling law. Like many physical parameters of polymers, rheological behavior of a gelling polymeric system can be also approximately described by power laws. Various power laws have been proposed and examined to explain or predict rheological features of gelling systems, especially for the sol-gel transition[3-14]. In the polymer industry the determination of the gelation threshold is an important issue to control the mechanical properties of a gelling system[15]. To study a gelling system, it is important to know how to determine its gel point. Traditionally, many researchers use the crossover of storage modulus G′(ω) and loss modulus G″(ω) as an indicator of the gel point. This method is simple and convenient, but not valid in general. A valuable contribution to this area is attributed to the researches of chemical and physical gelations in WINTER’s laboratory[5-10, 13]. The frequency independence of the loss tangent in the vicinity of the gel point has been widely examined for chemical and physical gels and has also been employed to determine the gel point[2, 6-14]. The definition of the gel point by this power law is excellent because a gelation variable loses its dependency on frequency and converges at the gel point. The experimental results have shown that this method is reliable for determination of the gel point such as the critical gelation time and the critical gelation temperature[6-14].
In our systems, the method employed to form a gel was adding a nonsolvent to the solvent mixture and/or low processing temperature. A mixture of good solvent with a nonsolvent was used as the solvent mixture for PAN[16]. The solvent mixture can be considered as a not-so-good solvent suitable to make PAN gel with the proper control of the nonsolvent amount. The gel structure and properties can be revealed through studying the rheology behavior of the gels.
In this paper, the work focuses on how the sol-gel transition of PAN solutions can be determined rheologically when the temperature governs the physical gelation and what the scaling law is for the gelation in the gelling system.
2 Experimental
2.1 Materials
PAN copolymers (acrylonitrile : itaconic acid=98?2) were provided by Shanghai Institute of Synthetic Fiber with a viscosity-average molecular mass = 7.8×104 g/mol. DMSO (analytically pure) was purchased from Shanghai Wulian Chemical Industry Co. Ltd. Deionized water was used.
2.2 Preparation of PAN solution
A certain amount of PAN copolymers were dispersed respectively in the mixture of DMSO and H2O. The resulting slurry was swollen at 50 ℃ for 8 h. Subsequently, it was stirred at 70 ℃ for 8 h to produce a homogeneous viscous PAN solution. Four such PAN solutions with a polymer concentration (mass fraction) of 23% and H2O contents (mass fraction) of 0, 2%, 4% and 6% were used, respectively. The solutions were deaerated in a vacuum drying oven at 70 ℃ for the removal of air bubbles and then kept at this temperature for 24 h before the rheological experiments.
2.3 Characterization
The dynamic rheometer (ARES-RFS, made by TA, U.S.) equipped with two parallel plates was employed to measure the storage modulus G′ and loss modulus G″ as a function of angular frequency ω at a certain temperature. The temperature control was done with a thermostatic bath within ±0.1 ℃ of the preset value. The parallel plate on which samples were placed was 25 mm in diameter. A thin layer of paraffin oil was applied to protect the sample from dehydration or evaporation and thus minimized errors. All frequency sweep measurements were carried out by using a variable temperature step of 2 ℃/min from 70 ℃ to a low temperature at which the PAN solutions could gel. Depending on the viscoelastic properties of each sample, the linearity of dynamic viscoelasticity was ensured by applying the suitable amplitude of shear strain. The data on the temperature dependence of the viscoelastic behavior were obtained from the frequency sweep data measured at various temperatures by a cooling procedure. Before the test at each temperature, all the samples were kept at that temperature for a reasonable time to eliminate the effect of the thermal history. A new solution was chosen for each new test in order to avoid the memory effects.
3 Results and discussion
3.1 Theoretical description of gelation rheology
Traditionally, many people use the crossover temperature of storage modulus G′ and loss modulus G″ at a constant frequency as a measure of the gel point. Since the crossover is frequency-dependent, however, this way to determine the sol-gel transition temperature was not used. As confirmed by a number of experiments [6-7, 9-11, 13-14], the frequency independence of loss tangent, is able to give an accurate determination of the gel point.
CHAMBON and WINTER[5] first reported a power-law behavior for the shear modulus over a wide range of shear frequencies of a permanently gelling system. They found experimentally for poly-dimethyl siloxane samples a scaling law G′(ω)=G″(ω)∝ω1/2 at the gel point and later generalized it to:
G′(ω)~G″(ω)∝ωn, 0
or
G″(ω)/G′(ω)=tanδ=tan(nπ/2) (2)
For all gelling systems, experimentally, n is found not to be constant, but it has a value between 0 and 1[6-14]. On the other hand, in the theoretical field, MARTIN et al[3-4] predicted that n is between 2/3 and 1. Therefore, it seems that there is no universal value of n, probably because n is related to the specific nature of each gelling system.
3.2 Determination of sol-gel transition temperature
In this work, we also examine this method for determining the sol-gel transition temperatures of the PAN solutions. Since tan δ loses its dependence on frequency and converges at the gel point, the gel point is determined from a multifrequency plot of tan δ vs gelation time, cross-linking degree, concentration, or temperature depending on which variable governs the gelation process. In this work, since the gelation variable was temperature, we plotted tan δ against temperature in order to decide the critical temperatures by this method. An example for this kind of plot is shown in Fig.1 for the PAN solution with H2O content of 4%. In Fig.1, each kind of symbol represents tan δ measured at a certain angular frequency ω. The angular frequency ranged from 0.1 rad/s to 39.8 rad/s. One can observe the convergent point of all tan δ vs temperature curves, which is then defined as the sol-gel transition temperature tgel (=39.6 ℃ in this case). Simultaneously, the value of tan δ at the gel point provides the information about the scaling exponent n of Eqn.(2), and then we directly obtained n=0.86 by using the relation of tan δ = tan (nπ/2) at the gel
Fig.1 Loss tangent tan δ as function of temperature for PAN solution with 4% H2O at various angular frequencies (Above tgel, from top to bottom, ω=0.100, 0.398, 1.000, 3.980, 10.000 and 39.800 rad/s. tgel is the gel point.)
point. Another example for this kind of plot is shown in Fig.2 for the PAN solution with H2O content of 6%. For other samples, we were able to accurately determine their sol-gel transition temperatures in this way within reasonable experimental error. The results are shown in Table 1. These results indicate that the scaling exponent n is independent of the sol-gel transition temperature. The universal value of n(=0.86), which is independent of the sol-gel transition temperature, suggests that the critical PAN gels have the similarity in the fractal structure.
Fig.2 Loss tangent tan δ as function of temperature for PAN solution with 6% H2O at various angular frequencies (Above tgel, from top to bottom, ω=0.100, 0.398, 1.000, 3.980, 10.000 and 39.800 rad/s. tgel is the gel point.)
Table 1 Values of gelation parameters for PAN/DMSO/H2O solutions with different H2O contents
Now we know that n is a universal exponent for the PAN gels. This result is very significant because it suggests an important feature of the critical PAN gels, that the sol-gel transition takes place at the same viscoelastic distance from a completely viscous liquid (n=1) or from a completely elastic solid (n=0). Although the gel strength at the gel point is dependent on molecular mass, polymer concentration, and temperature, the same value of n suggests an equivalent viscoelasticity at the gel point and may also mean the formation of the similar fractal structure.
It is noticeable that the gel point increases with increasing H2O content in the solvent mixture. It is obvious that H2O favors molecular aggregation of PAN molecules in solvents of DMSO and H2O. H2O is a strong cohesive solvent owing to the strong hydrogen bonds and it strongly opposes any solute that weakens the water-water interactions. Hence water promotes the aggregation of PAN molecules[17]. It is recognized that the pendant nitrile groups in PAN are partially intermolecularly coupled by attractive intermolecular interaction. This aggregation actually causes physical crosslinking points for the system. In our system when H2O is evenly distributed in DMSO, the whole system can be viewed as a network consisting of these physical crosslinking points. Actually, due to the localization tendency of this aggregation, the whole system can be more possibly considered as a network of the local molecule aggregations. These local molecule aggregations can be viewed as supermolecules. It is recognized that the solution or gel of supermolecules is more sensitive to temperature than that of small molecules. The PAN solution with more H2O is more sensitive to temperature and gels easily on cooling. Therefore, the gel point of the PAN solution with more H2O is higher.
4 Conclusions
Four PAN solutions with different H2O contents were prepared. The studies of the dynamic viscoelastic properties were conducted as a function of temperature for these PAN solutions. The sol-gel transition temperatures were able to be accurately determined by the frequency independence of loss tangent. The gel points of PAN solutions increase with increasing H2O content. It is obvious that H2O favors molecular aggregation of PAN molecules in solvents of DMSO and H2O. The scaling exponent n(=0.86) at the gel point was confirmed to be universal for PAN gels, which was independent of temperature, suggesting the similarity of the fractal structure in the critical PAN gels.
References
[1] NIJENHUIS K T. Thermoreversible networks-Viscoelastic properties and structure of gels-Introduction [J]. Advances in Polymer Science, 1997, 130: 1-12.
[2] WINTER H H, MOURS M. Rheology of polymers near liquid-solid transitions [J]. Advances in Polymer Science, 1997, 134: 165-234.
[3] MARTIN J E, ADOLF D, WILCOXON J P. Viscoelasticity of near-critical gels [J]. Physical Review Letters, 1988, 61: 2620-2623.
[4] MARTIN J E, ADOLF D. The sol-gel transition in chemical gels [J]. Annual Reviews in Physical Chemistry, 1991, 42: 311-339.
[5] CHAMBON F, WINTER H H. Stopping of crosslinking reaction in a PDMS polymer at the gel point [J]. Polymer Bulletin, 1985, 13: 499-503.
[6] WINTER H H, CHAMBON F. Analysis of linear viscoelasticity of a crosslinking polymer at the gel point [J]. Journal of Rheology, 1986, 30(2): 367-382.
[7] CHAMBON F, WINTER H H. Linear viscoelasticity at the gel point of a crosslinking PDMS with imbalanced stoichiometry [J]. Journal of Rheology, 1987, 31(8): 683-697.
[8] VILGIS T A, WINTER H H. Mechanical selfsimilarity of polymers during chemical gelation [J]. Colloid and Polymer Science, 1988, 266: 494-500.
[9] SCANLAN J C, WINTER H H. Composition dependence of the viscoelasticity of end-linked poly(dimethylsiloxane) at the gel point [J]. Macromolecules, 1991, 24(1): 47-54.
[10] IZUKA A, WINTER H H, HASHIMOTO T. Molecular weight dependence of viscoelasticity of polycaprolactone critical gels [J]. Macromolecules, 1992, 25(9): 2422-2428.
[11] KJONIKSEN A L, NYSTROM B. Dynamic light scattering of poly(vinyl alcohol) solutions and their dynamical behavior during the chemical gelation process [J]. Macromolecules, 1996, 29(22): 7116-7123.
[12] PEYRELASSE J, LAMARQUE M, HABAS J P, BOUNIA N E. Rheology of gelatin solutions at the sol-gel transition [J]. Physical Review E, 1996, 53(6): 6126-6133.
[13] MOURS M, WINTER H H. Relaxation patterns of nearly critical gels [J]. Macromolecules, 1996, 29(22): 7221-7229.
[14] KOIKE A, NEMOTO N, WATANABE Y, OSAKI K. Dynamic viscoelasticity and FT-IR measurements of end-crosslinking α,ω-dihydroxyl polybutadiene solutions near the gel point in the gelation process [J]. Polymer Journal, 1996, 28(11): 942-950.
[15] RICHTER S. Recent gelation studies on irreversible and reversible systems with dynamic light scattering and rheology-a concise summary [J]. Macromolecular Chemistry and Physics, 2007, 208: 1495-1502.
[16] NAGASHIMA N, MATSUZAWA S, OKAZAKI M. Syndiotacticity-
rich poly(vinyl alcohol) fibers spun from N-methylmorpholine-N-
oxide/water mixture [J]. Journal of Applied Polymer Science, 1996, 62(10): 1551-1559.
[17] PICULELL L, THURESSON K, LINDMAN B. Mixed solutions of surfactant and hydrophobically modified polymer [J]. Polymers for Advanced Technologies, 2001, 12: 44-69.
(Edited by HE Xue-feng)
Foundation item: Project(2006CB606505) supported by the National Basic Research Development Program of China; Project(50333050) supported by the National Natural Science Foundation of China; Project(07DJ14002) supported by the Shanghai Fundamental Theory Program; Project(20020255010) supported by the Specialized Research Fund for the Doctoral Program of Higher Education
Received date: 2008-06-25; Accepted date: 2008-08-05
Corresponding author: PAN Ding, Professor; Tel: +86-21-62199065; E-mail: ding pan@dhu.edu.cn
- Rheological behavior during thermotropic gelation of polyacrylonitrile concentrated solutions
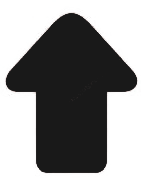