J. Cent. South Univ. Technol. (2008) 15(s1): 130-134
DOI: 10.1007/s11771-008-331-1
Challenging multiphase complex fluids for applications
XU Yuan-ze(许元泽)1, 2
(1. Department of Macromolecular Science, Fudan University, Shanghai 200433, China;
2. Key Laboratory of Molecular Engineering of Polymers of Ministry of Education,
Fudan University, Shanghai 200433, China)
Abstract:
Modern processing technology is calling the scientific understanding of dynamic processes, where the science of complex fluids plays a central role. We summarize our recent efforts using the generic approaches of multi-scale physics of complex fluids on apparently irrelevant processes, i.e. the mixing of polymer blends, the processing of thermoplastic(TP) toughened thermosetting(TS) composites using phase separation of TP in TS, as well as the enhanced oil recovery using polymer soft gel. It is emphasized that the thorough physical understanding in multi-scales of time and space through the joint efforts of experiment and theory in each scale is the key issue for the modeling of various processes.
Key words:
1 Introduction
The concept “complex fluid” denotes not only the matters, which cover very broad range from life to engineering materials behaving between solid and liquid, but also an approach to dealing with these fluids, differing from the way of phenomenological rheology. Indeed, challenging the complexity represents the history of rheology. We are still in the process of understanding the “complexity” of these fluids[1-2].
The splendid modern technology is calling the scientific understanding of the dynamic processes, where today’s structural rheology—the science of complex fluids should play a central role. Complexity may arise from the viscoelasticity of fading memory, from the multi-phase with interfacial energy or from the structuring due to gelation and the anisotropy from structural orientation. The structuring and the interactions behave the multi-scale nature in broad time-space range, as summarized in Table 1, where we summarize our recent efforts on apparently irrelevant applications in polymer blends[3-11], thermoplastic toughened thermosetting composites[12-16] as well as enhanced oil recovery by polymer soft gel[17-21], using the generic approaches of multi-phase complex fluids.
The coupling among these effects and the coupling of these effects with deformation and flow greatly complicate the already complex picture[1-2]. A lot of challenges are still under way, which will be discussed later as well.
2 Constitutive model of polymer blends
The melts of immiscible polymer blends are emulsions with two or more polymer phases, which usually form mesoscopic dispersive structures, from spherical or ellipsoidal droplets to bi-continuous morphology. It is of scientific and technological significance to form a rheological constitutive equation connecting the mesoscopic interfacial structure and macroscopic properties. If both phases are Newtonian fluids, the emulsion theory based on drop dynamics works, where the interfacial dynamics including drop deformation/breakup/merging can be analyzed. The component viscoelasticity is originated from the relaxation of entangled polymers in microscopic scales. One challenge to extend the model to the viscoelastic emulsion was the incorporation of component viscoelasticity to the multi-phase boundary conditions. The irreversible thermodynamic theory with Hamiltonian mechanical frame enjoys the additivity of the contributions of polymer viscoelasticity and interfacial energy at free energy level. Based on this, the rheological constitutive equations relating mesoscopic structures for polymer blends are established. Different effects of droplet dynamics, polymer conformations, component viscoelasticity, high concentration effect and interfacial rheological properties are considered[3-4]. The coupling between different mesoscopic structures is fulfilled. ?To verify the models connecting macro- and meso-scales, an experimental platform, a four-roll mill rheometer is con-
Table 1 Multi-scale approaches with application examples
structed for the systematic study of mesoscopic droplet dynamics, including the drop deformation, break-up and merging[6-11]. The accuracy of the constitutive models is justified by the experimental data in mesoscopic drop dynamics as well as macroscopic rheological functions in near linear range.
The deviations were found in the non-linear ranges of strong viscoelastic(VE) systems and large viscoelastic deformations. For example, the VE drops do not deform and relax as fast as expected: they do not breakup so easily as the Newtonian drops under capillary force; also, they are not easy to merge through squeezing critical film between drops. After repeated stretches, a VE drop may not be separated into two, rather form a dumbbell-like structure and merging finally without critical film collapse. This phenomenon, which we call as BSB (“bead-string-bead”) merging, is a VE relaxation dominant one[8-10]. This is a typical example that VE is strongly coupled with interfacial dynamics. As a matter of fact, for high VE blends, the contributions of interfacial energy are very limited. The rheological behaviors are dominated by VE components and their entangled network with complex morphological domains separated by interface.
The constitutive equations are integrated into the simulation of injection molding. A simulation for the full process of injection molding is established[5]. By integrating the constitutive model of polymer blends into three dimensional finite element simulation algorithm, it is possible to predict the mesoscopic structure and the macroscopic properties simultaneously. The approach realized the connection between mesoscopic structures and macroscopic properties and established a foundation for the further development of polymer blend processing to the step of morphology and property control, in the less coupled near linear range though.
3 Morphology control of TP/TS composites– phase separation approach
Improving the toughness of thermosetting resins (TS) through cure induced phase separation (PS) of thermoplastics (TP) is a promising approach with appropriate control of the phase separated morphological structure in certain space/time scales. For example, in the so called “ex-situ” processing of compression moulding, a morphology gradient and, thus, the modulus gradient from carbon fiber surface to TP mixed resin layer are formed through phase separation and diffusion processes simultaneously. The cure induced phase separation process proceeds in wide time/temperature window. The process was detected in situ by means of dynamic rheology and optical microscopic observations[12-16].
The morphology evolvement closely relates to the change of rheology, which is found to obey the critical gel theory of gelation. The theory claims that a random crosslinking process will form a percolated fractal network at the gel point. This causes the correlation of power index of rheological function to the fractal dimension of the network—a simple correlation of micro-/macro-[2,13,15].
The driving force of PS may be ascribed to the change of Flory-Huggins interaction constant χ by curing and the morphology evolution can be explained and simulated based on field theory applying to phase separation kinetics (e.g. Model B of Cahn with time dependent Ginzburg-Londau equation). This is a mesoscopic theory with microscopic details in χ.
An inversed optical microscope system allowing long-term observation of micro-morphology with high resolution at high temperature was established in our laboratory, which can successfully characterize the onset and evolvement of the phase separation process in various TP/TS systems. This allows direct correlation of structure—rheology—time—temperature data. Various TP/TS systems were investigated, including up and low critical separation temperature (UCST/LCST) systems and the systems with complex cure reaction kinetics.
Multi-critical gel transitions were found, which correspond to different gelation mechanisms: the gelation at phase separation being the result of entanglement of TP macromolecules, the gelation at the chemical gel point of crosslinking of thermosetting oligomers and the glass transition of TP phase during the post cure stage. The relaxation exponents of critical gels at different cure stages relate to the fractal dimensions of the critical gels of different network structures[16].
It was found that the cure induced phase separation time/temperature dependence can be well described by the Arrhenius equation[13-14, 16]. The phase separation activation energy Ea keeps constant with the variations of TP content, TP molecular weight, curing rate and phase separation detecting means, while varies with the chemical environments and the time/temperature dependence of the cure reaction. The change of Ea was elucidated in view of molecular interaction energy density for the UCST systems. It was shown that Ea values decrease with the deterioration of miscibility of the blends. With all these, TTT (time temperature transformation) diagram with PS information was established, which can provide intellectual structural control and processing profile of TP/TS products[16]. The morphology evolvement of compression moulding in processing window may be simulated by the modified phase separation model B of Cahn and Ginzburg-Londau with diffusion controlled TP concentration gradient.
4 Soft gel for EOR
Since the “oil crises” in 1970’s, this limited resource has warned us more and more frequently up to 21st century. Polymer flooding for enhanced oil recovery (EOR) already became the largest Non-Newtonian fluid process of economic significance. However, the mechanism of “how and what polymer works” is still to be clarified. Historically, polyacrylamide based systems finally gained most application. What is the essential reason and what are the future systems? The evolution of polymer application in EOR has been closely related to the evolution of rheological understanding. The concept evolution from viscosity ratio, elongational viscosity, viscoelasticity and hydrodynamic retention in the flow through porous media, interfacial viscoelasticity, depletion effect up to the association thickening, soft gelation, etc., brings the polymer flooding towards more difficult reservoirs of higher temperature, higher salinity and higher formation inhomogeneity. Our recent work on the relation of structure-rheology-application for the associated PAM and PAM soft gel approved that the multi-scale understanding of polymer flooding is essential for future development[17-21].
For example, in both AHPAM and weak gel approaches, various types of crosslink, the kinetics of gelation and the gel behaviors must be taken into account in the design EOR operations, but the control of inter- molecular cross-linking is decisive.
Hydrophobic group modified PAM chains have been introduced by means of copolymerization for solution thickening. Studies on structure-rheology relations of AHPAM systems reveal that starting from the semi-dilute concentration range, the presence of interchain liaisons leads to apparent viscosities much greater than those of the corresponding PAM. It is suggested that the concept of critical association concentration should be related to the transition from semi-dilute to entangled region rather than the start concentration of association. These solutions show shear thickening, rheopexic, and thixotropic behaviors, which may be explained in terms of the balance between inter- and intrachain liaisons and their effects on chain dimensions, while the temporary hydrophobically associating network can be destroyed when applying a critical shear stress[18]. It is important to control not only the association degree but also the random or blocky distribution of the hydrophobic units as well as the bulkiness of hydrophobes. The fact that the apparent elongational viscosity of AHPAM in converging flow decreases with flow rate reflects the intrinsic feature of the association: the association due to van de Waals force is a short range interaction, which once breaks down in strong flow and then recovers slowly. The thickening by entropy elasticity and the thinning by the association breaking down work together, resulting in the decreasing elongational viscosity with stretch rate. In terms of weaker viscoelasticity in strong flow and hard to control the intra/inter molecular associates the function of polymer association on EOR may be limited[17-18].
Soft gel, weak gel and colloidal gel are similar terms to describe the crosslinking system yet flowing. The use of water-soluble polymers coupled with proper concentration of cross-linkers has become a common practice in EOR. In such practice a solution containing the polymer and cross-linkers is injected in desired zones and allowed sufficient time to set into a three dimensional gel. In recent years, these gels are not only used in injection wells to divert the flow and to shut off the flow of water in oil production wells, but also used more substantially as enhanced polymer flooding. There are a number of gelling systems available for treatment of low temperature reservoirs. However, gels that can tolerate the harsh conditions of elevated temperatures and high salinity and divalent cations, which commonly present in deeper reservoirs, are limited.
For severe formation conditions, organic cross-linked HPAM gels remain viable to higher temperature, permit control of the gelation delay, and display good injectivity even when the residence time approaches the gelation time.
To describe the gelation process of weak gel and apply weak gel for the industrial practice of tertiary oil recovery, the classical branching theories of Flory show limitation because of the limited ability to distinguish the intra- and inter- molecular crosslinking. Three dimensional Monte Carlo simulations were carried out to obtain the growth of average molar mass and gel growth images[19]. Here flexible polymer is modeled as random flying chain in 3D. Each cell/step corresponds to a polymer segment with a probability to crosslink with other segments. The inter- and intra-crosslinks may be distinguished naturally. It is found that the initial polymer concentration is critical for the gel formation. The relationship between the speed of gelation and the concentration of crosslink agent was also simulated. The predicted dependency of the gel time upon the concentration of crosslink agent agrees well with the experimental data of viscosity versus time. Also, the polymer concentration effects are compared with those of the experiments. The polymer concentration changes inter/intra crosslink ratio. There is a critical concentration found by simulation, below which molecular weight growth to a limited value. Beyond the minimum concentration for gelation the polymer molecules are connected to clusters distributed in space inhomogeneously until forming a percolated network at gel point. The remarkable splitting of the molar mass distribution can be seen. The highest group is responsible for the network, while leaving the smaller groups or sol in the liquid. This is a typical state of weak gel. It is proved that Monte Carlo simulated mass averaged molar mass well agrees with experimental data of complex viscosity. At the concentration below the semi-dilute range, there is a time period for intermolecular cross linking through diffusion to begin. At high polymer concentration, the effect of concentration is insignificant because intermolecular gelation is always probable.
By analyzing the group conversion versus the conversion of the cross-linked macromolecules, it is revealed that the gelation progress is at the early stage of the reaction kinetics near the gel point[20]. With the rise of polymer concentration, the viscosity of the reaction system increases correspondingly in an exponential way, which is the characteristic of first-order reaction. The critical gel theory can be well employed to analyze the viscoelasticity data and determine the gel point and critical gel strength for the systems. Based on the dismutation scheme and experimental data, a rheological models of complex viscosity is introduced to describe the gelation process[20]. It is also found that by using some cross-linker some colloidal gel systems may be formed where two phases of sol and gel with characteristic dimension are clearly seen and the rheology will change, differing from the “random” gelation scheme of fractal type[21].
The non-linear rheological behaviors are more complicated for gel systems. Shear thinning and shear thickening, rheopexy and thixotropy become severe as the gelation proceeds. The viscosity curves in converging flow at various gelation stages are different from those of the AHPAM systems. This is related to the different natures of associated and chemical crosslinks.
It should also be emphasized that the channel sizes of porous media in reservoir also cover multi-scales, the interactions with fluids could be hydrodynamic, interfacial slippage, the depletion effect down to the adsorption at molecular level. The compatibility of the gel to channel size also needs to be considered in engineering applications.
What are the future systems? From PAM systems, we learnt useful principles: high thickening effect, entropy elasticity, association (H-bonding or hydrophobic interaction), controllable crosslinking as well as low price/property ratio. An essential weak point of PAM is the temperature resistance or thermal hydrolysis of solutions and gels, to which attention should be paid in developing future systems. Also, we should be responsible for the environment issues of crosslinking systems and long-term effects in polymer treated oil fields, to which much efforts should be made.
5 ConclusionsThe intelligent processing requires good control of optimized process and qualified structure and properties of end product. The rheology of complex fluids using the multi-scale approaches of soft matter physics may provide the scientific basis for this purpose. Here we emphasize three points of multi-scale approach: first, the problems are multi-scale in nature and different scales exhibit own dominate interaction and, thus, theoretical approaches; second, there is certain scale dominant a process and, third, there are possibilities of coupling or decoupling between scales.
For our case of polyblends, incorporation of interfacial dynamics with viscoelasticity in mesoscopic scale is the key issue, while low scales effects are reflected in interfacial and viscoelastic parameters. In the near-linear range the approach works quite well, while there is still challenge when getting into non-linear range of large shearing and strong viscoelasticity, where the decoupling of factors may be complex.
The target for toughening TP/TS composite is to control the phase separation morphology in processing window of time/temperature. The field theory and the critical gel theory in mesoscopic scale are sufficient to describe the physics of phase separation, while the structural effect on PS can be ascribed into interaction parameter, which is related to the chemical structures in molecular scale.
Polymer solution/gel for EOR is a typical example of multi-scale problem. Both fluids and media have multi-scale natures in structure and interactions. The further development of multi-scale approaches shall not only clarify the mechanism, but also apply for engineering design, including the match of fluid structure with pore structure.
It is emphasized in all cases that the thorough physical understanding in multi-scale of time and space through the combining efforts of theory and experiment in each scale is the key issue for the modeling of various processes.
AcknowledgementAll co-authors in reference literatures are sincerely appreciated.
References
[1] XU Yuan-ze. Frontiers and trends of rheology for complex fluids [M]. Beijing: Science Press, 2006: 315-323. (in Chinese)
[2] XU Yuan-ze. Gelation rheology and applications for complex fluids [C]// Proc of VIII National Congress on Rheology. Jinan: Shandong Univ Press, 2006: 32-34. (in Chinese)
[3] YU Wei, ZHOU Chi-xing, BOSMINA M. Theory of morphology evolution in mixtures of viscoelastic immiscible components [J]. Journal of Rheology, 2005, 49(1): 215-236.
[4] YU Wei, ZHOU Chi-xing, XU Yuan-ze. Rheology of concentrated blends with immiscible components [J]. Journal of Polymer Science (Part B): Polymer Physics, 2005, 43(18): 2534-2544.
[5] YAN B, ZHOU H, LI D. Numerical simulation of the filling stage for plastic injection moulding based on the Petrov–Galerkin methods [J]. Journal of Engineering Manufacture, 2007, 221(10): 1573-1577.
[6] XU Yuan-ze, WU Yu-zhe, YANG Jian-mao. Hydrodynamic and thermodynamic effects in phase inversion emulsification process of epoxy resin in water [J]. Chinese Journal of Polymer Science, 2006, 24(2): 155-161.
[7] YANG Jian-mao, XU Yuan-ze HU Yun-tao. The meso-scale investigation of multiphase fluids—Four-roll mill rheometer and its application [J]. Chinese Polym Bulletin, 2007, 4: 17-22.
[8] YANG Jian-mao, XU Yuan-ze. Coalescence of two viscoelastic droplets connected by a string [J]. Physics of Fluids, 2008, 20(4): 043101-1-9.
[9] YANG Jian-mao, XU Yuan-ze. Viscoelastic effect on drop coalescence—Bead-string-bead merging [J]. Acta Polymerica Sinica, 2008(8): 734-740. (in Chinese)
[10] YANG Jian-mao, XU Yuan-ze. Merging of viscoelastic droplets [C]// Proc of VIII national congress on rheology. Jinan: Shandong Univ Press, 2006: 178-184. (in Chinese)
[11] WU You-jun, YU Wei, ZHOU Chi-xing, XU Yuan-ze. Shape evolution of a single liquid crystal droplet immersed in an isotropic matrix under transient and steady flow [J]. Physical Review E, 2007, 75: 041706-1-9.
[12] ZHANG Xiu-juan, YI Xiao-su, XU Yuan-ze. The time/temperature relationship during phase separation of different thermoplastic modified thermosetting systems [J]. Acta Polymerica Sinica, 2007(8): 725-730. (in Chinese)
[13] ZHANG Xiu-juan, YI Xiao-su, XU Yuan-ze. Cure-induced phase separation of epoxy/DDS/PEK-C composites and its temperature dependency [J]. Journal of Applied Polymer Science, 2008, 109: 2195-2206.
[14] ZHANG Xiu-juan, YI Xiao-su, XU Yuan-ze. The effect of chemical structure on phase separation time/temperature dependencies in some thermoplastics modified thermoset systems [J]. Acta Polymerica Sinica, 2008(6): 583-591. (in Chinese)
[15] ZHANG Xiu-juan, XU Yuan-ze. Rheological and morphological study of the phase separation and gelation process of TP modified TS systems [C]// Proc of VIII National Congress on Rheology. Jinan: Shandong Univ Press, 2006: 138-144 (in Chinese)
[16] ZHANG Xiu-juan, YI Xiao-su, XU Yuan-ze. Phase separation time/temperature dependence of thermoplastic modified thermosetting systems [J]. Frontiers of Chemical Engineering in China, 2008, 2(3): 276-285.
[17] CAO Xu-long, LI Zheng-quan, ZHANG Kun-ling, WANG Gang, XU Yuan-ze. Multi-scale rheological perspective to polymer solutions and gels in EOR [J]. J Cent South Univ Technol, 2007, 14(s): 232-237.
[18] SUN Huan-zhang, ZHANG Kun-ling, CHEN Jing, ZENG Shen-wen, PING Zhen-hua, XU Yuan-ze. Effect of hydrophobic association on structure and rheological behavior of polyacrylamide aqueous solutions [J]. Acta Polymerica Sinica, 2006, 9(6): 810-814.
[19] YANG Jian-mao, CAO Xu-long, ZHANG Kun-ling, QIU Feng, XU Yuan-ze. Monte Carlo simulation of the gelation process of the aqueous polymer weak gel [J]. Chem J Chinese Univ, 2006, 27(3): 579-582. (in Chinese)
[20] ZHANG Kun-ling, LI Zheng-quan, LIU Qun, CAO Xu-long, XU Yuan-ze. The rheological analysis of gelation kinetics for partial hydrolyzed polyacrylamide weak gel [J]. Acta Polymerica Sinica, 2006(3): 518-522. (in Chinese)
[21] WANG Gang, XU Yuan-ze, FAN Xue-jing, CAO Xu-long, LIU Kun, ZHANG Ji-chao. Rheology and texture change of aqueous gelation systems [J]. Chem J Chinese Univ, 2008, 29(9): 1-4. (in Chinese)
(Edited by CHEN Wei-ping)
Foundation item: Project(20490224) supported by the National Natural Science Foundation of China; Project(2003CB615604) supported by the Major State Basic Research and Development Program of China; Project supported by Shengli oil field, SINOPEC Petrochemical Co. Ltd.
Received date: 2008-06-25; Accepted date: 2008-08-05
Corresponding author: XU Yuan-ze, Professor; Tel: +86-21-65643836; E-mail: yuanzexu@fudan.edu.cn
- Challenging multiphase complex fluids for applications
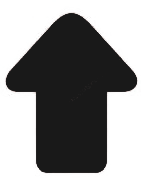