- Abstract:
- 1 Introduction▲
- 2 Experimental▲
- 3 Results and discus...▲
- 4 Conclusions▲
- References
- Figure
- Fig. 1 XRD pattern of cassiterite
- Fig. 2 Particles size distribution of cassiterite
- Fig. 3 Flotation recovery of ~10 μm SnO2 as a function of pH in absence and presence of collectors
- Fig. 4 Adsorption of SHA on cassiterite surface as a function of pH in absence and presence of TBP
- Fig. 5 Relationship between electrophoretic mobility and pulp pH with or without collectors
- Fig. 6 Infrared spectrum of cassiterite
- Fig. 7 Infrared spectra of SHA and TBP
- Fig. 8 Infrared spectrum of cassiterite after action of SHA
- Fig. 9 Infrared spectrum of cassiterite after action of TBP
- Fig. 10 Infrared spectrum of cassiterite after action of SHA mixed with TBP
- Fig. 11 Adsorption model of salicylhydroxamic acid on cassiterite surface
- Fig. 12 Coadsorption model of salicylhydroxamic acid and tributyl phosphate on cassiterite surface
J. Cent. South Univ. (2012) 19: 1711-1717
DOI: 10.1007/s11771-012-1197-9
Adsorption mechanism of mixed salicylhydroxamic acid and tributyl phosphate collectors in fine cassiterite electro-flotation system
QIN Wen-qing(覃文庆), REN Liu-yi(任浏祎), XU Yang-bao(徐阳宝), WANG Pei-pei(王佩佩), MA Xi-hong(马喜红)
School of Minerals Processing and Bioengineering, Central South University, Changsha 410083, China
? Central South University Press and Springer-Verlag Berlin Heidelberg 2012
Abstract:
Two reagents including salicylhydroxamic acid (SHA) and tributyl phosphate (TBP) were tested as collectors either separately or together for electro-?otation of fine cassiterite (<10 μm). Subsequently, the ?otation mechanism of the fine cassiterite was investigated by adsorbance determination, electrophoretic mobility measurements and Fourier transform infra-red (FT-IR) spectrum checking. Results of the ?otation experiments show that with SHA as a collector, the collecting performance is remarkably impacted by the pulp pH value as the ?oatability of cassiterite varies sharply when the pH changes, and flotation with SHA gives distinct maximum at about pH 6.5. Additionally, the ?oatability of cassiterite is determined by using SHA and TBP as collectors. The range of pulp pH for good ?oatability is broadened in the presence of TBP as auxiliary collector, and the utilization of TBP improves the recovery of cassiterite modestly. Moreover, the optimum pH value for cassiterite ?otation is associated with adsorbance. The results of FT-IR spectrum and the electrophoretic mobility measurements indicate that the adsorption interaction between the collectors and the cassiterite is dominantly a kind of chemical bonding in the form of one or two cycle chelate rings due to the coordination of carbonyl group, hydroxamate and P=O group to the metal tin atoms, where the oxygen atoms contained in carbonyl group, hydroxamate and P=O group of the polar groups have the stereo conditions to form ?ve-membered rings. In addition, the adsorption interactions of SHA and TBP on the surfaces of cassiterite are also dominated by means of hydrogen bonds.
Key words:
1 Introduction
Flotation of fine particles has become particularly important in recent years as advances in grinding, allowing low grade mineral deposits to be economically exploited [1-2]. Particle collection by air bubbles is regarded as the heart of froth flotation operation. Flotation performance depends on several key factors, such as particle size and surface chemistry of minerals. Several flotation technologies have been developed, aiming at increasing bubble-particle collision efficiency, either by decreasing bubble size or by increasing apparent particle size [3-4]. Due to the complexity of some ore bodies, it has become necessary to research fine particles flotation in order to improve mineral liberation [5]. Therefore, fine particles flotation has gained significant attention over the last decade.
The natural semi-conductor cassiterite, SnO2, has been proven to be one of the most difficult minerals to float. Many of the reported studies have focused on achieving selectivity between cassiterite and other minerals present in ore. Cassiterites are mainly found in late-stage granitic intrusions, as alluvia, placer and detrital deposits. Associated ganges including shales, arkosites, granites, granophyres and felsites could not be separated perfectly from the cassiterite during gravity concentration or froth flotation. Gravity separation is usually used for cassiterite separation from gangue [5-6], but the fine cassiterite particles (<20 μm) are hard to recover by gravity separation, then flotation is considered in cassiterite separation. A new method must be explored and used for the recovery of fine cassiterite particles.
Flotation reagents are the critical technique in the flotation separation process [6-9]. The objective for a collector in the flotation process is to create the best conditions for the air bubble attaching to the mineral surfaces. The adsorption of surfactants on mineral surfaces plays an important role in many industrial processes, particularly in froth flotation [10-11]. Over the last 30 years, many approaches have appeared on dealing with the determination of the solid surface free energy on the basis of electrophoretic mobility and infra-red (FT-IR) spectra measurements [12-16]. Generally, soaps and amines are effective but nonselective collectors. However, reagents containing hydroxamate groups are excellent collectors which hold the promise of being selective for cassiterite. SHA is one such reagent that is used for cassiterite flotation. Hydroxamate RCO(NHOH) as a fatty acid derivative, salts of which are chelating type of chemical reagents and are extensively used in the flotation of minerals due to their strong and selective complexation with a number of metal ions [17-19]. The non-ionic hydroxamic acid molecule can be adsorbed on cassiterite by a combination of physical and specific forces. Either SHA alone or in combination with TBP can be used in fine cassiterite flotation, and the same grade and recovery requirements can be achieved. Furthermore, when SHA mixed with TBP is used in fine cassiterite flotation, the amount of SHA can be reduced by half.
The purpose of the present work is to understand the adsorption mechanism of the mixture of SHA and TBP in fine cassiterite flotation. The electro-flotation, infrared spectroscopy, electrophoretic mobility, ultraviolet spectroscopy studies using fine cassiterite have been carried out. The interaction of hydroxamic acid with chemically inert cassiterite has been systematically investigated. In this work, the results of these investigations are discussed and the role of mixed collectors in fine cassiterite flotation is delineated.
2 Experimental
2.1 Materials
The high-grade cassiterite concentrate used in the experiments was obtained by gravity separation of a tin ore from Gaofeng Mining Co. Ltd. in Guangxi Autonomous Region, China. The concentrate was further purified by removing traces of magnetic impurities using magnetic separator. CaCO3 was removed by adding dilute hydrochloric acid solution (1%, mass fraction) and stirring for about a week. SiO2 was removed using shaking table. X-ray diffraction (XRD) result is shown in Fig. 1. The peaks of any impurity are not detected, which indicates that SnO2 is successfully obtained with high purity. Chemical analysis of the concentrate sample shows that it contains 94% SnO2, which is deemed sufficiently pure for research purposes. Particle size analysis of the sample is shown in Fig. 2. It can be seen that cassiterite less than 10 μm takes up about 95% in this sample.
SHA with 99% purity used as a collector was bought from Shanghai Jingchun Reagent Limited Company, and TBP (analytically pure) used as an assistant collector was bought from Changsha. Other chemicals employed in the test were of analytical reagent grade. All the experiments were carried out with distilled water only.
Fig. 1 XRD pattern of cassiterite
Fig. 2 Particles size distribution of cassiterite
2.2 Electro-flotation
A single bubble flotation tube made of Plexiglas was a modified Hallimond tube. The H2 bubbles produced from cathode screen of the single bubble flotation tube were used for carrying the fine cassiterite particles. The O2 bubbles produced from anode were separated by PTFE diaphragm and exported from latex tube connected above the anode. Electro-flotation tests were conducted in this special self-made Hallimond tube (100 mL in volume) at a constant ionic strength of 1% NaSO4 (mass fraction), while the pulp density was 1%. The mineral sample was conditioned with the collector reagent for 5 min in a tumbling machine with a magnetic stirrer bar (1 cm) at 1 000 r/min, followed by flotation for 8 min using H2 bubbles. In each test, firstly, the sample (1 g) was added in the Bunsen beaker with about 90 mL NaSO4 solution, and then dispersed with ultrasonic wave for 10 min. The pulp pH was adjusted to 7 using 1% NaOH solution and 1% H2SO4 solution, and then the collectors of SHA and TBP were added in the pulp. After stirring for 5 min, the pulp was transferred into the self-made electro-flotation tube with a peristaltic magnetic stirrer. By fixing the electro-flotation tube, connecting the power, turning on DC power supply, regulating current, and then electrifying for 1 min, the H2 bubbles were introduced. And conditioned for further 1 min, the cassiterite particles were removed by the rising H2 bubbles and the flotation was carried out. After 8 min, the floated materials were recovered, filtered, dried, weighed and analyzed.
Unless otherwise mentioned, the pH values stated were those measured before the experiments.
2.3 Infrared spectroscopy
In order to characterize the nature of the interactions between the collectors and fine cassiterite particles, the infrared spectra of collectors as well as the samples with or without collectors pretreated were measured by the KBr technique. Fourier transform infra-red (FT-IR) spectra of the samples were recorded with Nicolet Model Nexus 670 (USA) instrument using KBr pellet, at a resolution of 4 cm-1.
2.4 Particle size analysis
To prove the pre-supposed range of grain size, particle size analysis of the sample was determined on Master sizer 2000 (Malvern/ England) instrument.
2.5 Measurement of electrophoretic mobility
The electrophoretic mobility of cassiterite was determined on Coulter Delsa440sx Instrument (Backmen-coulter/USA). The measurements were conducted by taking 0.5 g of mineral in 100 mL of surfactant solution. NaOH (1%, mass fraction) solution and H2SO4 (1%, mass fraction) solution were used for adjusting pulp pH value. An average of four measurements was taken for each mobility point.
2.6 Ultraviolet spectroscopy
All the adsorption experiments were carried out with SHA reagent only or a combination of SHA and TBP. Each test was carried out by equilibrating 1 g cassiterite with 100 mL SHA (or a combination of SHA and TBP) solution of known concentration. Equilibration at a desired temperature was performed in a temperature controlled water bath. After the completion of pre-defined conditioning period, the solids were separated by high-speed centrifugation, operated for 5 min at 5 000 r/min. The absorbency of the solution was determined on a Tu-1870 UV–Visible spectrophotometer (Beijing Puxin General Equipment Limited Liability Company, China). The amount of SHA adsorbed on cassiterite was calculated by Eq. (1). All the experiments were repeated in quadruplicate and the reproducibility of the data was checked.
(1)
where Γ is adsorption concentration of mineral (mol·L-1·cm-2); c0 is the initial concentration of collector (mol/L); c is the residual concentration of collector (mol/L); V is the volume of flotation solution (mL); m is the mass of mineral (g); S is the specific surface area of mineral (cm2/g).
3 Results and discussion
3.1 Electro-flotation
The recovery of fine cassiterite particles as a function of pH in the 1% NaSO4 solution is shown in Fig. 3 for no reagents, SHA only, SHA in combination with TBP. The results show that cassiterite does not have natural floatability. It can be recovered under the action of SHA or SHA mixed with TBP at pH range from 6.0 to 8.0, but outside this range the recovery decreases sharply. The highest recovery is obtained at about pH 6.5. A better flotation response can be achieved when SHA and TBP are used as collectors, as shown in Fig. 3. TBP can be used for cassiterite flotation as an auxiliary collector, which not only helps to recover cassiterite, but also makes dosage of SHA reduce and costs of reagents cut. The adsorption mechanism will be explored in the following tests.
Fig. 3 Flotation recovery of ~10 μm SnO2 as a function of pH in absence and presence of collectors
3.2 Adsorption
The adsorption of SHA on cassiterite is also studied at different pH values, the results of which are shown in Fig. 4. Maximum adsorption amount of SHA at cassiterite/water interface is obtained at a pH around 6.5 and this maximum is more pronounced when TBP is used as an auxiliary collector. At higher or lower pH values the adsorption decreases sharply. The results are in agreement with the flotation tests presented above.
3.3 Electrokinetics
Figure 5 gives the electrophoretic mobility of cassiterite with no reagent, in the presence of SHA and SHA mixed with TBP under different pH conditions. The iso-electric point (IEP) of cassiterite sample in the presence of different electrolytes alone occurs at about pH 5.8. This disagrees with the general range of IEP values obtained by several researchers for cassiterite samples of different origins [6, 20-21]. The possible reason is that SO42- absorbed on the surfaces of cassiterite influences the IEP of cassiterite. The adsorption of anion on the cassiterite surface helps to improve the exchange adsorption of collector. On equilibration with SHA, the IEP of the mineral shifts towards more acidic pH to about 4.5 and an increase in negative charge on the mineral is also observed. This phenomenon can be defined as the action of an anionic collector (SHA). The electrophoretic mobility of cassiterite becomes smaller in the presence of SHA, that is to say, SHA makes the negative electrophoretic mobility of cassiterite more negative in 4.5-10 of pH range. The anionic collector SHA overcomes the electrostatic repulsion and is absorbed on the cassiterite surface, indicating that there is a stronger force (chemical bonding force).
Fig. 4 Adsorption of SHA on cassiterite surface as a function of pH in absence and presence of TBP
Fig. 5 Relationship between electrophoretic mobility and pulp pH with or without collectors
Again, the electrophoretic mobility of cassiterite is measured after the assistant reagent TBP is added in the cassiterite pulp. It is found that the IEP of the mineral shifts towards more alkaline with pH to 4.6 and an increase in positive charge on the mineral is also observed. Utilization of TBP increases the positive charge on the cassiterite surface. The chemical adsorption of TBP on the cassiterite surface occurs.
3.4 Infrared spectroscopy
To further investigate the interaction mechanism of SHA and TBP on minerals, influences of SHA and TBP on the FT-IR spectra of cassiterite are studied. The FT-IR spectra of cassiterite, SHA and TBP are presented in Figs. 6 and 7. The FT-IR spectra of cassiterite treated with SHA, TBP, a combination of SHA and TBP are indicated in Figs. 8-10.
Fig. 6 Infrared spectrum of cassiterite
Fig. 7 Infrared spectra of SHA and TBP
The spectrum of cassiterite (Fig. 6) agrees well with the standard spectrum of SnO2. The characteristic Sn—O band at 647.965 cm-1 can be seen. The IR spectrum of free SHA shown in Fig. 7 exhibits the typical N—H stretching band at 3 288.036 cm-1, and the —OH vibrating absorption bands in the wavenumber range of 2 944.769-3 261.037 cm-1. Other distinct absorption peaks are observed at 2 000-1 620 cm-1 (frequency doubling from benzene ring), 1 617.984 cm-1 (C=O vibrating), 744.388 cm-1 (C—H vibrating, benzene ring 1, 2 are substituted), 1 245.789 cm-1 (C—O stretching vibration), 904.451 cm-1 (N—O stretching vibration). These values agree with the published literatures [6, 9].
Fig. 8 Infrared spectrum of cassiterite after action of SHA
Fig. 9 Infrared spectrum of cassiterite after action of TBP
Fig. 10 Infrared spectrum of cassiterite after action of SHA mixed with TBP
Two bands in the range of 2 856.059-2 969.839 cm-1 and 1 378.854-1 467.563 cm-1 (Fig. 7) are assigned to the stretching vibration and bending vibration absorption of C—H in CH3 and CH2 groups. The stretching vibration absorption peak of P=O band is noticed at 1 258.94 cm-1, and the strong adsorptions at 1 114.95 cm-1, 1 029.43 cm-1, 907.47 cm-1 are attributed to the P—O—C stretching vibration, indicating that P=O and P—O—C bonds are the polar groups of TBP, while the hydrocarbon chain is nonpolar group.
For the IR spectra of cassiterite treated with or without SHA (shown in Fig. 8 and Fig. 6, respectively), a chelated characteristic absorption peak appears at 3 343.1 cm-1, indicating that the chelate exists on the surface of the cassiterite. Two weak absorptions at 1 685.48 and 1 725.978 cm-1 are attributed to the C=O vibration. The characteristic peak of cassiterite at 647.965 cm-1 moves to 690.391 cm-1, which indicates that the chemical adsorption of SHA generates on cassiterite surface. Other faint characteristic peaks at low bands also move to the higher bands.
A band at 809.68 cm-1 (Fig. 9) of an absorption peak is observed due to bending vibration of O—P=O bond, which is in great accordance with those reported in previous literature [22]. The characteristic peak of cassiterite has a shift to the higher bands. The faint absorptions at low bands (below 700 cm-1) may be caused by the impurity element included in the sample or the presence of alkyl groups.
From the infrared spectrum of cassiterite treated with SHA and TBP shown in Fig. 10, the P=O bond at 1 258.94 cm-1 disappears, and characteristic absorption peak of P—O—C appears at 1 096.70 cm-1, indicating that both P=O and P—O bonds change sharply, and the oxygen atom in P=O bond takes part in coordination. The characteristic absorption peaks in range of 2 944.769-3 261.037 cm-1 and at 1 617.984 cm-1 have a shift to higher bands, indicating that the —N—OH and C=O bond in SHA work with Sn, which results in the disappearing of hydroxyl and the weakening of C=O bond. The characteristic peak of cassiterite at 647.965 cm-1 moves to 709.99 cm-1. The faint absorptions around 650 cm-1 are attributed to the associating of hydroxyl in SHA. A new complex may be produced by the coordination of P=O, —P—OH, —N—OH, C=O and —Sn— bands at 1 376.25 cm-1, 1 199.74 cm-1, 913.75 cm-1, 709.99 cm-1 and 687.69 cm-1. SHA and TBP are absorbed on the surface of cassiterite successively, resulting in a new complex chelate produced on the surface of cassiterite. The adsorption on cassiterite is improved and enhanced, hydrophobic property of cassiterite increases, and the floating ratio of cassiterite is improved finally.
3.5 Interaction mode of salicylhydroxamic acid and tributyl phosphate on cassiterite surface
One kind of possible adsorption model of SHA and the coadsorption model for SHA and TBP on the surface of cassiterite can be signified in the following models shown in Figs.11 and 12, respectively.
Fig. 11 Adsorption model of salicylhydroxamic acid on cassiterite surface
Fig. 12 Coadsorption model of salicylhydroxamic acid and tributyl phosphate on cassiterite surface
In the polar groups of the collectors, —OH of the hydroxy group and —NHOH of the hydroxamate group may dissociate oxygen anions, which possess strong coordination capacity for the formation of covalent bonds to tin atom on cassiterite surface. Additionally, the oxygen atom in the carbonyl group CO has lone-pair electron with high negative value of net charge, which presents that the atom has the possibility of bonding with cassiterite. Meanwhile, it has the natural conditions to form five-membered ring. Combined with the analysis of electrophoretic mobility and IR spectra as well as the results of flotation experiments, the interaction mechanism of the collector (SHA) and cassiterite can be concluded that five-membered chelate ring may be formed on the surface of mineral through chemical bonding (Fig. 11); meanwhile, hydrogen bond may also be formed on the cassiterite surface by the groups of OH and CONHOH.
In addition, the interaction mechanism of the collectors (SHA and TBP) and cassiterite can be concluded that hydrogen bond may also be formed by the nitrogen atom in —NH and +HOX in TBP (Fig. 12), making more adsorption of the auxiliary collector on the chelate molecule, thus improving the hydrophobicity of chelate and flotation recovery of cassiterite.
4 Conclusions
1) The electro-?otation recovery of fine cassiterite (<10 μm) is tested using SHA and TBP as collectors separately or together. The results of ?otation experiments show that the collecting performance is remarkably impacted by the pulp pH value and flotation with SHA gives distinct maximum at about pH 6.5. Additionally, the ?oatability of cassiterite is determined by using SHA and TBP as collectors. The range of pulp pH for good ?oatability is broadened in the presence of TBP as an auxiliary collector, which improves the recovery of cassiterite modestly. The optimum pH range for cassiterite ?otation is 6.5-7.0.
2) The nature of interaction between reagents (SHA and TBP) and cassiterite is studied using ultraviolet spectroscopy, electrokinetic and infrared spectroscopy. Adsorption amount is more observed in neutral or faintly acid pH and is almost invariant within the faintly acid range studied, i.e. pH 6.5-7.0. The adsorption interaction between the collectors and cassiterite is dominantly a kind of chemical bonding in the form of one or two cycle chelate rings. The oxygen atoms contained in carbonyl group, hydroxamate and P=O group of the polar group have the stereo conditions to form ?ve-membered rings. In addition, the adsorption interactions of SHA and TBP on the surfaces of cassiterite are also dominated by means of hydrogen bonds.
3) The adsorption model of SHA and the coadsorption model of SHA and TBP on the surface of cassiterite are explored.
References
[1] GEORGE P, NGUYEN A V, JAMESON G J. Assessment of true flotation and entrainment in the flotation of submicron particles by fine bubbles [J]. Minerals Engineering, 2004, 17(7/8): 847-853.
[2] WATERS K E, HADLER K, CILLIERS J J. The flotation of fine particles using charged microbubbles [J]. Minerals Engineering, 2008, 21(12/13/14): 918-923.
[3] MIETTINEN T, RALSTON J, FORNASIERO D. The limits of fine particle flotation [J]. Minerals Engineering, 2010, 23(5): 420-437.
[4] GONTIJO C D, FORNASIERO D, RALSTON J. The limits of fine and coarse particle flotation [J]. Canadian Journal of Chemical Engineering, 2007, 85(5): 739-747.
[5] CILEK E C, UMUCU Y. A statistical model for gangue entrainment into froths in flotation of sulphide ores [J]. Minerals Engineering, 2001, 14(9): 1055-1066.
[6] SREENIVAS T, PADMANABHAN N P H. Surface chemistry and flotation of cassiterite with alkyl hydroxamates [J]. Colloids and Surfaces A: Physicochemical and Engineering Aspects, 2002, 205(1/2): 47-59.
[7] JIANG Y R, ZHAO B N, ZHOU X H, ZHOU L Y. Flotation of diaspore and aluminosilicate minerals applying novel carboxyl hydroxamic acids as collector [J]. Hydrometallurgy, 2010, 104(1): 112-118.
[8] HU Y, LIU X, XU Z H. Role of crystal structure in flotation separation of diaspore from kaolinite, pyrophyllite and illite [J]. Minerals Engineering, 2003, 16(3): 219-227.
[9] WU X Q, ZHU J G. Selective flotation of cassiterite with benzohydroxamic acid [J]. Minerals Engineering, 2006, 19(14): 1410-1417.
[10] FUERSTENAU D W, PRADIP. Zeta potentials in the flotation of oxide and silicate minerals [J]. Advances in Colloid and Interface Science, 2005, 114: 9-26.
[11] CHEN G L, TAO D, REN H, JI F F, QIAO J K. An investigation of niobite flotation with octyl diphosphonic acid as collector [J]. International Journal of Mineral Processing, 2005, 76(1/2): 111-122.
[12] SOMASUNDARAN P, UNJAPPU J T K. In-situ investigation of adsorbed surfactants and polymers on solids in solution [J]. Colloids and Surfaces A: Physicochemical and Engineering Aspects, 1989, 37: 245-268.
[13] OZCAN O, BULUTCU A N. Electrokinetic, infrared and flotation studies of scheelite and calcite with oxine, alkyl oxine, oleoyl sarcosine and quebracho [J]. International Journal of Mineral Processing, 1993, 39(3/4): 275-290.
[14] HU Y H, XU Z H. Interactions of amphoteric amino phosphoric acids with calcium-containing minerals and selective flotation [J]. International Journal of Mineral Processing, 2003, 72(1/4): 87-94.
[15] LIMA R M F, BRANDAO P R G, PERES A E C. The infrared spectra of amine collectors used in the flotation of iron ores [J]. Minerals Engineering, 2005, 18(2): 267-273.
[16] VIDYADHAR A, RAO K H. Adsorption mechanism of mixed cationic/anionic collectors in feldspar-quartz flotation system [J]. Journal of Colloid and Interface Science, 2007, 306(2): 195-204.
[17] HU Y, WANG D, XU Z. A study of interactions and flotation of wolframite with octyl hydroxamate [J]. Minerals Engineering, 1997, 10(6): 623-633.
[18] ASSIS S M, MONTENEGRO L C M, PERES A E C. Utilisation of hydroxamates in minerals froth flotation original research article [J]. Minerals Engineering, 1996, 9(1): 103-114.
[19] YOON R H, NAGARAJ D R, WANG S S, HILDEBRAND T M. Benefication of kaolin clay by froth flotation using hydroxamate collectors [J]. Minerals Engineering, 1992, 5(3/5): 457-467.
[20] ZHU D, ZHU Y S. Research on surface electric property of cassiterite [J]. Non-ferrous Mining and Metallurgy, 1994(1): 19-22. (in Chinese)
[21] SHI D M. Cassiterite flotation and surface electric property [J]. Journal of Kunming University of Science and Technology: Science and Technology, 1987, (3): 38-45. (in Chinese)
[22] THOMAS L C,CHITTENDE R A. Characteristic infrared absorption frequencies of organophosphorus compounds: I. The phosphoryl (P—O) group [J]. Spectrochimica Acta, 1964, 20(3): 467-487.
(Edited by HE Yun-bin)
Foundation item: Project(50774094) supported by the National Natural Science Foundation of China; Project(2010CB630905) supported by the National Basic Research Program of China
Received date: 2011-10-19; Accepted date: 2012-04-14
Corresponding author: QIN Wen-qing, Professor, PhD; Tel: +86-731-88830884; E-mail: qinwenqing1@126.com
Abstract: Two reagents including salicylhydroxamic acid (SHA) and tributyl phosphate (TBP) were tested as collectors either separately or together for electro-?otation of fine cassiterite (<10 μm). Subsequently, the ?otation mechanism of the fine cassiterite was investigated by adsorbance determination, electrophoretic mobility measurements and Fourier transform infra-red (FT-IR) spectrum checking. Results of the ?otation experiments show that with SHA as a collector, the collecting performance is remarkably impacted by the pulp pH value as the ?oatability of cassiterite varies sharply when the pH changes, and flotation with SHA gives distinct maximum at about pH 6.5. Additionally, the ?oatability of cassiterite is determined by using SHA and TBP as collectors. The range of pulp pH for good ?oatability is broadened in the presence of TBP as auxiliary collector, and the utilization of TBP improves the recovery of cassiterite modestly. Moreover, the optimum pH value for cassiterite ?otation is associated with adsorbance. The results of FT-IR spectrum and the electrophoretic mobility measurements indicate that the adsorption interaction between the collectors and the cassiterite is dominantly a kind of chemical bonding in the form of one or two cycle chelate rings due to the coordination of carbonyl group, hydroxamate and P=O group to the metal tin atoms, where the oxygen atoms contained in carbonyl group, hydroxamate and P=O group of the polar groups have the stereo conditions to form ?ve-membered rings. In addition, the adsorption interactions of SHA and TBP on the surfaces of cassiterite are also dominated by means of hydrogen bonds.
- Adsorption mechanism of mixed salicylhydroxamic acid and tributyl phosphate collectors in fine cassiterite electro-flotation system
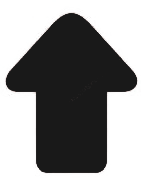