J. Cent. South Univ. Technol. (2010) 17: 801-806
DOI: 10.1007/s11771-010-0559-4
Lyapunov-Krasovskii functional based power system stability analysis in environment of WAMS
LI Ting(李婷), WU Min(吴敏), HE Yong(何勇)
School of Information Science and Engineering, Central South University, Changsha 410083, China
? Central South University Press and Springer-Verlag Berlin Heidelberg 2010
Abstract:
In order to analyze power system stability in environment of WAMS (wide area measurement system), a new steady state stability model with time-varying delay was proposed for power system. The factors of exciter and power system stabilizer with delay were introduced into analytical model. To decrease conservativeness of stability analysis, an improved Lyapunov-Krasovskii functional was constructed, and then a new delay-dependent steady state stability criterion for power system, which overcomes the disadvantages of eigenvalue computation method, was derived. The proposed model and criterion were tested on synchronous- machine infinite-bus power system. The test results demonstrate that Lyapunov-Krasovskii functional based power system stability analysis method is applicable and effective in the analysis of time delay power system stability.
Key words:
power system; steady state stability; time-varying delay; power system stabilizer;
1 Introduction
With the development of wide area measurement system (WAMS) in power system in recent years, new feature of power system stability problem emerges [1-4]. WAMS consists of phasor measurement unit (PMU) and phasor data concentrator (PDC). PMU continuously streams time-stamped measurement data to PDC at central locations where a range of power grid monitoring and control computer are running. To synchronize PMUs at different locations, GPS system is used to provide precise time in 1 μs. WAMS was used to analyze power system performance such as damping interarea oscillation [5-6], load shedding [7], and state estimation [8].
Though PMU can provide accurate information of state variables of power system in the same time coordinates and give the feasibility of wide area control for power system, the information should be delivered from measurement location to control center, and then processed as input signals of control devices. Apparently, time delay exists in the delivery and communication of data and has a significant influence on power system stability. Further study of power system stability model in environment of WAMS became interests of electrical engineers and scholars in the world [9-13].
In conventional model of power system stability analysis [14-15], the influence of time delay is not considered. ROMMES and MARTINS [16] proposed eigenvalue calculation method to compute small signal stability for larger-scale power system. AZBE et al [17] presented energy function method to analyze multimachine system stability with a unified power flow controller. MARSZALEK and TRZASKA [18] used singularity-induced bifurcations to discuss power system stability. However, in environment of WAMS, time delay cannot be neglected because, according to the theory of time delay control system [19-20], inherent delay of electrical devices and delivery delay of control signal may cause power system unstable. Moreover, these delays are time-varying, which enhance the difficulty of dynamic stability analysis of power system. Frequency domain analysis cannot be applicable to power system with time-varying delay. Eigenvalue computation method can only be used to analyze power system stability without delay or with constant delay [21-23]. Therefore, new stability methods considering time delay influence are needed to be studied.
In the work, a new steady stability analysis model of power system with time-varying delays is constructed. Based on the proposed model, an improved Lyapunov- Krasovskii functional is used to derive a new delaydependent steady stability criterion, which extends eigenvalue computation method in conventional steady state analysis. Numerical examples of synchronous- machine infinite-bus power system validate the correctness of the proposed model and criterion.
2 Problem description
Dynamic equations of generator can be given below:
(1)
(2)
(3)
(4)
(5)
where δ is the rotor angle of generator, rad; ωs is the synchronous angular speed of generator, rad/s; ω is the rotor angular speed of generator, rad/s; m is the mechanical starting time, s; Pm is the mechanical active power, kW; Pe is the electrical active power, kW; D is the damping coefficient; is the q-axis voltage of generator, kV;
is the q-axis transient voltage of generator, kV; Ef is the excitation voltage, kV; xd is the d-axis synchronous reactance of generator, Ω; xq is the q-axis synchronous reactance of generator, Ω;
is the d-axis transient reactance of generator, Ω;
is the d-axis transient short circuit time constant of generator, s; Id is the d-axis circuit of generator, A; Iq is the q-axis circuit of generator, A; Ud is the d-axis voltage of generator, kV; and Uq is the q-axis voltage of generator, kV.
Exciter dynamic equation with time-varying delay can also be given below:
(6)
where Ke is the exciter gain constant; Te is the exciter time constant, s; d(t) is the time delay; Uref is the reference bus voltage, kV; Ut is the generator terminal voltage, kV; ΔU=Uref-Ut; UPSS is the control voltage of power system stabilizer (PSS), kV. In the environment of WAMS, Uref is usually retrieved from remote measurement point.
PSS dynamic equations with time-varying delay can also be given below:
(7)
(8)
where KPSS is the gain constant of power system stabilizer; T1, T2 and T3 are the time constants of power system stabilizer, s; y1 and y2 are the state variables of PPS.
Eqs.(1)-(8) are steady stability analysis models of power system with time-varying delays in the environment of WAMS.
3 Delay-dependent stability analysis
Define at equilibrium point
where ?δ is the rotor angle deviation; Δω is the rotor angular speed deviation; ΔE′q is the q-axis transient voltage deviation; ?Ef is the excitation voltage deviation; ?y1 is the deviation value of the 1st state and ?y2 is the deviation value of the 2nd state variable of PSS. The next steady stability analysis criterion will be derived in the following section.
3.1 Model transformation
At equilibrium point, generator, exciter and PSS dynamic equations can be transformed into the following equations:
(9)
(10)
(11)
(12)
(13)
(14)
(15)
To demonstrate basic principle of the proposed model, synchronous-machine infinite-bus power system was chosen as the object to be studied. Then, the following is true.
(16)
where U0 is the infinite bus voltage, kV; Ux is the x-axis terminal voltage of generator, kV; Uy is the y-axis terminal voltage of generator, kV; xe is the transmission line reactance between generator and finite bus; Ix is the x-axis terminal circuit of generator, A; and Iy is the y-axis terminal circuit of generator, A.
By Eqs.(9)-(16), steady stability analysis model of power system with time-varying delays can be transformed into:
(17)
where d(t) is the time delay, and it is time-varying with lower and upper bound, and its derivative has also upper bound. State matrix A is given below:
(18)
where
and time delay state matrix Ad is given below:
(19)
where
3.2 Improved Lyapunov-Krasovskii functional
For the proposed steady stability model, delay- independent criterion can be derived by using the following Lyapunov-Krasovskii functional:
(20)
where h is upper bound of time delay d(t); and Z1 and Z2 are positive matrices to be determined. Apparently, because delay-independent criterion dose not consider the influence of time delay, there is much more conservativeness for power system steady stability problem. So, in recent years, much attention has been focused on delay-dependent criterion which is used for time delay system analysis, and the following Lyapunov- Krasovskii functional is usually chosen.
(21)
where Z1, Z2 and Z3 are positive matrices to be
determined. However, for the derivative of
the term
is enlarged to be
hence, the other term
is neglected. Therefore, Eq.(21)
may lead to conservativeness. Based on the above analysis, an improved Lyapunov-Krasovskii functional is constructed to derive a new delay- dependent steady state stability criterion for power system:
(22)
3.3 Steady state stability criterion
Lemma 1: For given matrix
where
the following three inequalities are equivalent:
(23)
Theorem 1: Given scalars h>0 and μ, steady state of the power system in Eq.(17) will be asymptotically stable if there exist matrices:
>0, i=1, 4, 5
≥0, i=2, 3
and
such that the following LMI (linear matrix inequality) holds:
<0 (24)
where
Proof: From the Leibniz-Newton formula, the following equations are true of any matrices N, S, and M with appropriate dimensions:
(25)
(26)
(27)
where
Calculating the derivative of V3(t, xt) along the solutions of system in Eq.(17) and adding the left side of Eqs.(25)-(27) into it yield:
≤
(28)
Since Z4>0 and Z5>0, the last three parts in Eq.(28) are all less than 0. So, if
<0, which is equivalent to Eq.(24) by lemma 1, then there exists
<
for a sufficiently small ε>0 such that steady state of the power system in Eq.(17) is asymptotically stable.
Remark 1: Based on the improved Lyapunov- Krasovskii functional in Eq.(22), a new delay-dependent steady state stability criterion for power system is derived. Because both time-delay influences on stability
criterion and the relative term
in our Lyapunov-Krasovskii functional are considered, the proposed steady state stability criterion has less conservativeness than Eqs.(20) and (21). The proposed criterion for power system in Eq.(17) is in the form of linear matrix inequalities and can be solved by Matlab LMI Toolbox.
Remark 2: Conventional model of power system steady state stability analysis can be described as and by solving
stability performance can be analyzed. Our proposed model in this work extends the conventional model by adding
which introduces time delay influence and is very appropriate for steady state analysis in the environment of WAMS.
4 Numerical example
Parameters of generator, exciter, and PSS are given in Tables 1 and 2. The time delay model of exciter and PSS in Eqs.(6)-(8) was chosen. Time delay of data delivery from reference node to central location in exciter and inherent time delay of PSS were considered.
Table 1 Parameters of generator
Table 2 Parameters of exciter and PSS
Assume that 0≤d(t)≤h and ≤μ, where μ is upper bound of time delay rate. Operation point was given by δ0=0.884 7 rad, ω0=1 rad/s and U0=1 kV. xd=0.132 Ω,
0.324 Ω, xq=0.617 Ω, xe=0.901 Ω. By Theorem 1, the upper bound of time-varying delay h is given in Table 3.
Another Lyapunov-Krasovskii functional Eq.(21) in expression was chosen to compute steady state stability criterion. When the upper bound of time delay rate μ is equal to 0, 0.2, and 0.4, respectively, Eqs.(21) and (22) have the same results, but when μ is equal to 0.6, 0.8, and 1.0, Eq.(22) has better results than Eq.(21). This means
that by using the improved Lyapunov-Krasovskii functional, the proposed steady state stability criterion has less conservativeness when time delay changes violently and can provide more precise upper bound of time delay.
Table 3 Upper bound of time-varying delay (h)
According to conventional steady state stability method, the power system without considering time- varying delay will be stable if and only if the real parts of eigenvalues of state matrix are all negative. However, in the environment of WAMS, the conventional method is not appropriate and only the proposed steady state stability criterion can be used to analyze power system stability. The curves of state variables with respect to time for power system in Eq.(17) are given in Fig.1.
Fig.1 Power system stability analysis when h=0.119 7 s and μ=0.6: (a) Rotor angle deviation; (b) Rotor angular speed deviation; (c) q-axis transient voltage deviation; (d) Excitation voltage deviation; (e) Deviation value of the 1st state variable of PSS; (f) Deviation value of the 2nd state variable of PSS
From Fig.1, it can be noticed that when h=0.119 7 s and μ=0.6, deviations of rotor angle and rotor angular speed, which are main indexes of steady state stability of power system in conventional steady state stability method, can return to be zero. Other state variable deviation values can also return to be zero in a period of time. It means that when power system with wide area control equipments installed is subjected to any small signal disturbance, it can return to its initial operation state and is still stable after several seconds.5 Conclusions
(1) A new time-delay steady state stability model for WAMS assisted power system is constructed, which considers time-varying delay influence of the exciter and PSS and can precisely depict the mechanism of power system stability.
(2) A novel delay-dependent steady stability analysis criterion for power system with less conservativeness is derived by an improved Lyapunov- Krasovskii functional, which can overcome disadvantages of eigenvalue computation method and can be realized by using LMI toolbox.
(3) The proposed model and criterion are tested on synchronous-machine infinite-bus power system and simulation results prove the effectiveness of steady state analysis method in the environment of WAMS.
References
[1] PHADKE A G, de MORAES R M. The wide world of wide-area measurement [J]. IEEE Power and Energy Magazine, 2008, 6(5): 52-65.
[2] HAUER J F, MITTELSTADT W A, MARTIN K E, BUMS J W, LEE H, PIERRE J W, TRUDNOWSKI D J. Use of the WECC WAMS in wide-area probing tests for validation of system performance and modeling [J]. IEEE Transactions on Power Systems, 2009, 24(1): 250-257.
[3] XIE Xiao-rong, XIN Yao-zhong, XIAO Jin-yu, WU Jing-tao, HAN Ying-dao. WAMS applications in Chinese power systems [J]. IEEE Power and Energy Magazine, 2006, 4(1): 54-63.
[4] KUNDUR P, PASERBA J, AJJARAPU V, ANDERSSON G. Definition and classification of power system stability [J]. IEEE Transactions on Power Systems, 2004, 19(3): 1387-1401.
[5] HAUER A J F, TRUDNOWSKI D J, DESTEESE J G. A perspective on WAMS analysis tools for tracking of oscillatory dynamics [C]// IEEE Power and Energy Society General Meeting. New York: IEEE Power Engineering Society, 2007: 1-10.
[6] FAR H G, BANAKAR H, LI P, LUO C L, OOI B T. Damping interarea oscillations by multiple modal selectivity method [J]. IEEE Transactions on Power Systems, 2009, 24(2): 766-775.
[7] SEETHALEKSHMI K, SINGH S N, SRIVASTAVA S C. WAMS assisted frequency and voltage stability based adaptive load shedding scheme [C]// IEEE Power and Energy Society General Meeting. New York: IEEE Power Engineering Society, 2009: 1-8.
[8] LI Hong, LI Wei-guo. A new method of power system state estimation based on wide-area measurement system [C]// Proceeding of the 4th IEEE Conference on Industrial Electronics and Applications. New York: IEEE Industrial Electronics Society, 2009: 2065-2069.
[9] LA S M, BENEDICTIS M, BRUNO S, GROBOVOY A, BONDAREVA N, BORODINA N, DENISOVA D, GEMOND A J, CHERKAOUI R. Development of applications in WAMS and WACS: An international cooperation experience [C]// IEEE Power and Energy Society General Meeting. New York: IEEE Power Engineering Society, 2006: 892-906.
[10] WU H X, TSAKALIS K S, HEYDT G T. Evaluation of time delay effects to wide-area power system stabilizer design [J]. IEEE Transactions on Power Systems, 2004, 19(4): 1935-1941.
[11] OKOU F, DESSAINT L A, AKHRIF O. Power systems stability enhancement using wide-area signals based hierarchical controller [J]. IEEE Transactions on Power Systems, 2005, 20(3): 1465-1477.
[12] RAY S, VENAYAGAMOORTHY G K. Real-time implementation of a measurement-based adaptive wide-area control system considering communication delays [J]. IET Generation, Transmission and Distribution, 2008, 2(1): 62-70.
[13] DOTTA D, SILVA A S, DECKER I C. Wide-area measurements- based two-level control design considering signal transmission delay [J]. IEEE Transactions on Power Systems, 2009, 24(1): 208-216.
[14] RUEDA J L, COLOME D G, ERLICH I. Assessment and enhancement of small signal stability considering uncertainties [J]. IEEE Transactions on Power Systems, 2009, 24(1): 198-207.
[15] ABOYTES F, SANCHEZ F, CABRA A I M, CASTRO J E G. Dynamic stability analysis of the interconnected Colombia- Venezuela power system [J]. IEEE Transactions on Power Systems, 2000, 15(1): 376-381.
[16] ROMMES J, MARTINS N. Computing large-scale system eigenvalues most sensitive to parameter changes, with applications to power system small-signal stability [J]. IEEE Transactions on Power Systems, 2008, 23(2): 434-442.
[17] AZBE V, GABRIJEL U, POVH D. The energy function of a general multimachine system with a unified power flow controller [J]. IEEE Transactions on Power Systems, 2005, 20(3): 1478-1485.
[18] MARSZALEK W, TRZASKA Z W. Singularity-induced bifurcations in electrical power systems [J]. IEEE Transactions on Power Systems, 2005, 20(1): 312-320.
[19] XIAO Shen-ping, WU Min, SHE Jin-hua. Non-fragile delay- dependent H∞ control of linear time-delay system with uncertainties in state and control input [J]. Journal of Central South University of Technology, 2008, 15(5): 712-719.
[20] HE Yong, WU Min, SHE Jin-hua, LIU Guo-ping. Parameter- dependent Lyapunov functional for stability of time-delay system with polytopic uncertainties [J]. IEEE Transactions on Automatic Control, 2004, 49(5): 828-832.
[21] DU Zheng-chun, LIU Wei, FANG Wan-liang. Calculation of rightmost eigenvalues in power systems using the Jacobi–Davidson method [J]. IEEE Transactions on Power Systems, 2006, 21(1): 234-239.
[22] MA J, DONG Z Y, ZHANG P. Comparison of BR and QR eigenvalue algorithms for power system small signal stability analysis [J]. IEEE Transactions on Power Systems, 2006, 21(4): 1848-1855.
[23] YANG D, AJJARAPU V. Critical eigenvalues tracing for power system analysis via continuation of invariant subspaces and projected Arnoldi method [J]. IEEE Transactions on Power Systems, 2007, 22(1): 324-332.
Foundation item: Projects(60425310, 60974026) supported by the National Natural Science Foundation of China; Project(200805330004) supported by the Doctor Subject Foundation of China; Projects(NCET-06-0679) supported by Program for New Century Excellent Talents in University; Project(08JJ1010) supported by the Natural Science Foundation of Hunan Province, China
Received date: 2010-03-07; Accepted date: 2010-05-30
Corresponding author: LI Ting, PhD; Tel: +86-731-88830387; E-mail: litingcsu@163.com
(Edited by LIU Hua-sen)
- Lyapunov-Krasovskii functional based power system stability analysis in environment of WAMS
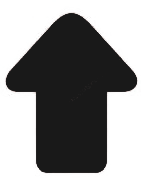