- Abstract:
- 1 Introduction▲
- 2 Field investigations▲
- 3 Results of laboratory a...▲
- 4 Discussion▲
- 5 Conclusions▲
- References
- Figure
- Fig.1 General pictures of inlet and inside of Fala tunnel: (a) Inlet of Fala tunnel; (b) Side-wall and roof of tunnel
- Fig.2 Appearance of side-wall of Fala tunnel covered by salt crystal and sulfate solution with very high concentration
- Fig.3 Whitish soft product of core sample selected near drainage in Fala tunnel
- Fig.4 White salt crystals in concrete sample cored from side wall of Fala Tunnel: (a) Floccule crystal on surface; (b) Crystal in pore of concrete
- Fig.5 Appearances of pore concrete by long-term water leaching role from side wall of Fala Tunnel: (a) Surface percolating zone; (b) Porous concrete core located percolating zone
- Fig.6 Tested results of distributions of sulfur and sodium elements of cored sample: (a) Sulfur element distribution on top-side; (b) Sodium element distribution on top-side; (c) Sulfur element distribution on down-side; (d) Sodium element distribution on down-side
- Fig.7 Tested results of distributions of calcium and silicon elements of cored sample: (a) Calcium element distribution on top-side; (b) Silicon element distribution on top-side; (c) Calcium element distribution on down-side;(d) Silicon element distribution on down- side
- Fig.8 Microanalysis of mushy substance taken from sample given in Fig.3: (a) SEM image; (b) EDS pattern of location of (a)
- Fig.9 X-ray diffractive spectrum of selected samples shown in Fig.3
- Fig.10 Microanalysis of surface substance taken from sample given in Fig.4: (a) SEM image; (b) EDS pattern of location of (a)
- Fig.11 Microanalysis of red substance taken from sample given in Fig.5: (a) SEM image; (b) EDS pattern of location of (a)
J. Cent. South Univ. Technol. (2011) 18: 881-888
DOI: 10.1007/s11771-011-0777-4
Deterioration of concrete in railway tunnel suffering from sulfate attack
LONG Guang-cheng(龙广成)1, XIE You-jun(谢友均)1, DENG De-hua(邓德华)1, LI Xiao-kun(李小坤)2
1. School of Civil Engineering and Architecture, Central South University, Changsha 410075, China;
2. Kunming Railway Bureau, Kunming 650011, China
? Central South University Press and Springer-Verlag Berlin Heidelberg 2011
Abstract:
For the sake of understanding the deterioration behavior of concrete in actual railway tunnel structures subjected to aggressive sulfate medium in practice, detailed field investigations and tested analysis on sprayed concrete linings of approximately 40-year-old railway tunnels in environments containing sulfate ion were carried out, respectively. The results show that the deterioration of concretes in the investigated area is serious, which involves complicated physicochemical process between the sulfate salt and concrete. Among them, the secondary sulfate minerals such as gypsum formation under very high concentration sulfate ion condition by accumulating and evaporation process dominate, followed by the crystallization of sulfate salt and formation of thaumasite.
Key words:
sulfate attack; concrete; railway tunnel; secondary sulfate minerals; salt crystallization;
1 Introduction
The external sulfate attack (ESA) is one of the main factors that cause the durability problem of concrete structure in service, therefore the sulfate attack on concretes has been greatly documented over the last several decades [1-3]. The sulfate attack to cement concrete is a complex process that involves the movement of sulfate ions through the pores by different transportation mechanisms. And the interaction of aggressive solution with some compounds of cement paste (AFm phases, hydrogarnet phases, unhydrated alumina phases and CH) to form expansive compounds (ettringite and gypsum) produces cracking, strength loss and softening of concrete [4-7]. The most common mineral products caused by sulfate attack are gypsum (CaSO4?2H2O) and ettringite (3CaO?Al2O3?3CaSO4? 31H2O) [8-9]. Thaumasite product is the third mineral caused by sulfate attack, which has been increasingly found in cement-based materials exposed to sulfates. Thaumasite is a calcium silicate carbonate sulfate hydrate (CaSiO3?CaCO3?CaSO4?15H2O) and can be found occurring alongside ettringite, gypsum or on its own [10-12]. ERLIN and STARK first reported its occurrence in concrete construction in sanitary sewer pipes and at the base of a core taken from an 11-year-old pavement. Since then, the thaumasite form of sulfate attack (TSA) has become recognized as a separate form of sulfate attack and has been found in different buildings of England, US, Canada, France, Switzerland, Swiss, Germany, Norway, Italy and other places [10]. The first case of concrete structure damage caused by thaumasite form in China was reported by MA et al [13] on the investigation of Bapanxia Hydraulic Power Plant in Western China in 2004. In other places of China, especially in southwest of China, deteriorations of concrete structures in practice due to sulfate attack were found gradually in recent years. However, the corresponding degrading behavior of concrete in actual structures has not been understood clearly until now.
In this work, the deterioration of concrete lining of more than 40-year-old railway tunnels located in southwestern region containing sulfate ion in groundwater and soil, was investigated to further uncover the degrading behavior and corresponding mechanism of actual railway tunnel structure.
2 Field investigations
In this investigation, several railway tunnels, including Fala tunnel and Bijiguan tunnel, located in Chuxiong city of Yunnan Province were inspected. And the concrete samples cored. These tunnels were built about at the beginning of 1965. This region locates in the southwestern region of Yun-Gui Altiplano, belonging to sub-torrid zone, and the year-average temperature is about 12 °C. With respect to engineering geological characteristics, the main rock includes the red sandstone, mud-based sandstone and gypsum mine in cretaceous. The red sandstone was also used to build the side-wall of tunnel. The Fala tunnel investigated in this work has a length of 2 100 m. Figure 1 is the general picture of Fala Tunnel at the present state. The vault (roof) of tunnel was supported by shotcrete and the side-wall was built by red sandstone and mortar or concrete. Serious damage of the whole tunnel including tunnel inlet and inside was observed though three larger scale repairs had been made in 1982, 1987 and 2000, respectively. And small scale repairs and maintenances are almost carried out each year according to the related records.
Fig.1 General pictures of inlet and inside of Fala tunnel: (a) Inlet of Fala tunnel; (b) Side-wall and roof of tunnel
In our inspection, it was in early January. The air temperature was 2-8 °C. Water occurred randomly at various locations of the side-walls and roof of the tunnels in the form of percolation, dripping and bedewing. The appearance within the tunnel is marvellous spectacle. Much white salt crystal was distributed in the roof and wall of the tunnel (see Fig.1 and Fig.2). One can also see that, from Fig.1 and Fig.2, many traces of concrete damage located in roof and side-wall existed. Various types of damage, including the swelling and flaking away of the concrete from the surfaces, were observed. It can be found that, besides the salt crystal on the surface, chemical reaction has taken place on the inner side. Near the drainage or other damp position, the cementitious substance with a hard state has changed into a soft, white mushy powder (see Fig.3). At those locations where there is humid, a light grey mud can be observed. Another typical deterioration of concrete is the loss of the hydrated products by the leaching role of ambient solution and the microstructure of concrete has changed from dense into porous. Pictures given in Fig.3, Fig.4 and Fig.5, which were taken from on-site and cored sample, represent the typical deterioration of concrete observed on-site. The concrete was seriously damaged.
Fig.2 Appearance of side-wall of Fala tunnel covered by salt crystal and sulfate solution with very high concentration
Fig.3 Whitish soft product of core sample selected near drainage in Fala tunnel
From the available technical documentation and related records, the deterioration of concrete by the sulfate salt attack occurred about one year after each repair by shotcrete. The shotcrete used to repair the tunnels consisted of one part of binder, and two parts of limestone chipping with size of 0.075-4.75 mm, and two parts of crushed limestone with size of 4.75-10 mm by mass fraction, with small quantities of chemical additives. The binder was Portland cement and ground granular blast furnace slag. This indicates that the repair materials composed of the above raw materials has no resistance to such serious sulfate attack.
Fig.4 White salt crystals in concrete sample cored from side wall of Fala Tunnel: (a) Floccule crystal on surface; (b) Crystal in pore of concrete
Fig.5 Appearances of pore concrete by long-term water leaching role from side wall of Fala Tunnel: (a) Surface percolating zone; (b) Porous concrete core located percolating zone
3 Results of laboratory analysis
Concrete samples have been cored carefully from the representative locations showing deterioration phenomena. Samples were classified according to typical appearances. Then, the samples were chosen for microscope or analytical investigation after some treatments such as cutting and drying. The corresponding analysis tests include scanning electron microscopy (SEM), X-ray diffractometry (XRD), energy dispersive X-ray spectroscopy (EDX) and electron probe microscopy (EPMA), which aim at determining the change of hydration products, uptake or change in the concentration of chemical components and element mappings on the investigated samples. And also the water sample from the drainage of the Fala tunnel was analyzed by chemical method.
3.1 Water sample analysis
Table 1 lists the analytical result of water sample from the drainage located in Fala tunnel.
Table 1 Result of water sample analysis (mg/L)
From the result listed in Table1, one can find that there are sulfate ion, calcium ion, magnesium ion, sodium ion and a small quantity of chloride ion in the water sample. The pH value of water is 6.5 and is almost neutral. This shows that the water sample includes aggressive mediums, such as sulfate, which might be one of the most important factors causing deterioration of concrete lining of tunnel. From the point of view of the concentration of sulfate ion in the solution, it is not extremely high. However, the sulfate ion in the pore solution of concrete can come up to a very high concentration or even crystallization process by evaporation role or accumulative process.
3.2 Typical appearance of deteriorative concrete core samples
Through the on-site investigation, it can be found that the main deterioration formations of concrete lining of Fala tunnel include three types, which are shown in Fig.3, Fig.4 and Fig.5, respectively. By combining the investigated results and related physical and chemical knowledge, it can be followed that the degradation of concrete results mainly from hydrated products change from sound or dense to porous through chemical reaction of aggressive salt by inleakage of ground water, salt crystallization, leaching role and carbonation.
From the photo shown in Fig.3, one can find that the sample is a soft substance with whitish and grey color and no cementitious characteristic. The damaged depth is up to 60 mm. This sample is located in drainage hereabout side-wall. It can be followed that the hydrated products of concrete changed greatly. The photo given in Fig.4 shows that there exists a lot of salts in the sample. And the white salt crystal was observed on the surface after the sample was placed in air-dry state one day. On can see that, from the photo in Fig.5, the sample is porous and substantial hydrated products are lost. This sample is located in the vicity of a cracked drainpipe of side-wall. Water flows from the inside of wall out to the surface of concrete. Obviously, the leaching role of groundwater results in the serious deterioration of concrete sample. Percolating groundwater along pathways through concrete is efficiently leaching alkalis and portlandite, leading to a leaching zone characterized by the reduction of portlandite while calcium silicate hydrate (C—S—H) phases are still present. During further leaching, all portlandite is removed and the pH value is reduced rapidly. And then the C—S—H phases are not stable anymore and start to dissolve, leading to a ‘corrosion zone’ consisting of a S—H residue [11,14]. During these leaching processes, the density of the hardened cement paste is reduced, accompanied by micro-cracking and therefore increasing the permeability properties (see Fig.5)
3.3 Micro-analysis of samples
3.3.1 EPMA analysis
In order to know the changes of characteristic elements and their distributions in the damaged concrete, the samples taken from the cored specimen shown in Fig.4 were tested with an EPMA-1600 apparatus. The elements such as sulfur, sodium, calcium and silicon were analyzed. The tested results are shown in Fig.6 and Fig.7.
From the results shown in Fig.6 and Fig.7, one can see that the highest content of sulfur element occurs at the top-side of the sample. And the content is also high within the region of 35 mm length of the sample, then the amount of sulfur element reduces with increasing the depth from the exterior. It is most noteworthy that the amount of sulfur element comes up to a high level again on the position of about 60 mm depth from the exterior. Moreover, a high content of sodium element in the range of 60 mm depth from the exterior of the sample is observed. However, from the results shown in Fig.7, distinct distribution changes of calcium and silicon elements in the sample do not occur. In addition, it is found that the carbonation depth tested by the phenolphthalein method is 60 mm. Generally, when the carbonation of concrete occurs, the sulfate ion (SO42-) in concrete will move toward non-carbonation zone from the carbonation area while sodium ion (Na+) will transfer from the non-carbonation zone to the carbonation zone. From the tested results of element distributions in the concrete sample, one can draw a conclusion that sulfur and sodium elements in the concrete come from the exterior of ambient water and soil which contain rich sulfate ions and sodium ions. And the carbonation of concrete can further contribute to the movement of the sulfate and sodium ions.
Fig.6 Tested results of distributions of sulfur and sodium elements of cored sample: (a) Sulfur element distribution on top-side; (b) Sodium element distribution on top-side; (c) Sulfur element distribution on down-side; (d) Sodium element distribution on down-side
3.3.2 SEM-EDS and XRD analysis
From the microstructure images and spectra shown in Fig.8, Fig.9, Fig.10 and Fig.11 tested by SEM, EDX and XRD, respectively, one can further understand the failure mechanism of concrete.
It can be found that, from the image shown in Fig.8(a), there are many stick-like substances in concrete. However, no CH crystal is observed in the sample and this compound is needed to preserve the integrity of CSH. Many club-shaped or needle crystals are embedded in the pulpy material with an open microstructure. A large number of needle crystals up to 0.3 μm in diameter and 3 μm in length, predominantly with parallel orientation and with smooth and even surfaces can be seen. The appearances of these crystals are very similar to those of thaumasite or ettringite as described in Refs.[3,11]. An EDS pattern of these crystals shown in Fig.8(b) indicates the presence of calcium, silicon, sulfur, aluminum, carbon, oxygen and some magnesium. A higher Si peak and a lower Al peak indicate that these needle crystals are predominantly thaumasite with a small quantity of ettringite.
Fig.7 Tested results of distributions of calcium and silicon elements of cored sample: (a) Calcium element distribution on top-side; (b) Silicon element distribution on top-side; (c) Calcium element distribution on down-side;(d) Silicon element distribution on down- side
Fig.8 Microanalysis of mushy substance taken from sample given in Fig.3: (a) SEM image; (b) EDS pattern of location of (a)
Fig.9 X-ray diffractive spectrum of selected samples shown in Fig.3
Fig.10 Microanalysis of surface substance taken from sample given in Fig.4: (a) SEM image; (b) EDS pattern of location of (a)
Fig.11 Microanalysis of red substance taken from sample given in Fig.5: (a) SEM image; (b) EDS pattern of location of (a)
In order to analyze the products shown in Fig.8, the sample selected from the same location was further tested by XRD. The XRD analysis result of the selected sample is shown in Fig.9, where it can be seen that, due to the deterioration, there is little trace of the products of normal cement hydration such as portlandite (CH) in the XRD trace. The trace indicates that the thaumasite crystalline phases as well as ettringite, gypsum, calcite crystallization exist in the sample. The XRD patterns of thaumasite and ettringite are similar, but both of them can be recognized when the XRD pattern is locally magnified. Another detected product of sulfate attack presented in the trace is calcite, which is formed from the carbonation of portlandite. This result further confirms that the concrete is greatly attacked by the chemical action of sulfate salt, which is one of the main causes resulting in serious deterioration of concrete in tunnel.
From the image shown in Fig.10(a), it can be found that the microstructure is very loose, which consists of some lager cluster-shaped or flake-shaped matters. An EDX pattern of this flake shown in Fig.10(b) indicates that this flake mainly includes sodium and sulfur. By combining the analysis of the appearance of white crystal covered on the surface of concrete and its microstructure, it can be followed that this flake is sodium sulfate crystal by the crystallization role of salt solution.
Obviously, based on the microscope analysis, it can be followed that the ingress of ambient sodium sulfate solution into concrete results in the degradation of concrete by the chemical action and physical crystallization, which includes the change of microstructures, cracking or scaling off of concrete.
In order to further understand the red substance almost covering the whole pore concrete, the powder scratched from the surface of porous concrete shown in Fig.5 was analyzed by SEM and EDX. The corresponding image is shown in Fig.11(a). From the image, one can see that there exists plenty of colloid- shaped material, which is composed of red substance. The EDS pattern shown in Fig.11(b) reveals that the main element consisting of colloid-shaped material is iron. Thus, it is deduced that this red substance is the deposit of Fe(OH)3, which results from the oxidation of low valence iron from the ambient ground water or soil.
4 Discussion
As mentioned above, the concrete in the investigated railway tunnel lining structures is seriously damaged by the sulfate attack. And the complicated physicochemical interactions between aggressive sulfate ions and cement hydration products of concrete occur, which form a plenty of deleterious secondary sulfate minerals including gypsum, ettringite, thaumasite and produced sulfate crystallization. Obviously, there is much corrosive medium such as sulfate ions in the investigated tunnels. According to this investigation, the aggressive sulfate ions in the concrete mainly come from two aspects. The first resource is the inleakage of abundant groundwater with sulfate solution into the concrete. Other sulfate is from the coal powder with sulfur, which scatters on the surface of the concrete when the train loading with coal runs through the tunnel. Due to appropriate temperature and relative humidity, the concrete has been damaged by serious sulfate attack [15]. The poor quality of concrete in actual tunnel lining structure is another important factor which should be mentioned. In the investigated tunnel, shotcrete linings are rather thin and inhomogeneous because the construction condition was limited 40 years ago, and low strength and high permeability concrete was used during construction. And the necessary waterproof measurements were almost not set. The transport velocity of ground water containing sulfate ions in actual tunnel structures concrete is rapid. Especially, the low relative humidity caused by frequent running through of train speedups the transport process and even accumulative or crystallization role of aggressive salt solution of concrete. Thus, the deteriorative process of concrete is accelerated.
The physicochemical processes between sulfate solution and concrete may differ from each other in different areas and thus the degradation process of concrete will also differ. For the concrete located at the bottom of lining layer which is long-term immersed into the ground water containing sulfate ions, the damage of concrete results from the formation of gypsum, ettringite and the decomposition of C—S—H [1,6]. However, this process will take a long time due to a relatively low concentration of sulfate ions in the surroundings. As for the concrete located on the surficial layer, for example, the concrete located near the damaged drainage, the reaction process involves more complicated mechanisms. The carbonate ions are available due to the dissolution of atmospheric CO2 in water and thaumasite is formed [10,12]. And then, the concrete begins to swell, flake and fall away in the shape of soft white particles without any cohesion, as seen in Fig.3. Among these physicochemical reactions, it is most noteworthy that the concentration of sulfate ions in the pores of concrete can reach a very high value due to gradual accumulating by the evaporation role, as seen in Fig.2. Under such high concentration sulfate ion conditions, the simple and typical chemical reactions between Ca(OH)2 and sulfate ions can occur and the secondary gypsum forms according to Eq.(1). The chemical reaction between sulfate ions and hydration product of cement is totally speeded up. Subsequently, the main hydration products in concrete, C—S—H gel, decomposes into Si gel following Eq.(2) when the alkalinity of the pore solution of concrete reduces to a certain extent as a result of Ca(OH)2 consumption. In addition, the crystallization process of sulfate salt on the surface of concrete will also occur as Eq.(3). Therefore, the deterioration of concrete is greatly accelerated by multiple aggressive actions. Such situation dominates the deterioration of most concrete in the investigated tunnel:
Ca(OH)2?CaSO4×2H2O? (1)
C—S—H+H2O?CaSO4×2H2O+SiO2 or Si gel (2)
2Na++10H2O?Na2SO4×10H2O (3)
5 Conclusions
1) The concrete in the investigated railway tunnel lining structures is seriously damaged by the sulfate attack. Complicated physicochemical interactions between aggressive sulfate ions and cement hydration products of concrete occur, which form a plenty of deleterious secondary sulfate minerals, including gypsum, ettringite, thaumasite and produce sulfate crystallization. The secondary gypsum formation under very high concentration sulfate ions condition caused by accumulating or surface evaporating role dominates, followed by the crystallization of sulfate salt as well as the formation of thaumasite.
2) It is very important for the design of underground constructions such as tunnel to set some waterproof steps and the drainage system and also consider its accessibility of maintenance operations. With respect to service life of tunnel structural concrete, the best solution would be to keep the concrete from direct contacting with the groundwater containing aggressive media.
References
[1] MEHTA P K. Sulfate attack on concrete—A critical review [C]// SKALNY J. Material Science of Concrete, Vol. III. American Ceramic Society, Westerville, USA, 1992: 105-130.
[2] NEVILLE A. The confused world of sulfate attack on concrete [J]. Cement and Concrete Research, 2004, 34(8): 1275-1296.
[3] ROMER M, HOLZER L, PFIFFNER M. Swiss tunnel structures: Concrete damage by formation of thaumasite [J]. Cement and Concrete Composites, 2003, 25: 1111-1117.
[4] HEARN N, YOUNG J F. w/c ratio, porosity and sulfate attack—A review [C]// MARCHAND J, SKALNY J. Materials Science of Concrete: Sulfate Attack Mechanisms. The American Ceramic Society, Westerville, USA, 1999: 189-205.
[5] SANTHANAM M, COHEN M D, OLEK J. Sulfate attack research–– whither now? [J]. Cement Concrete Res, 2001, 31: 845-851.
[6] SANTHANAM M, COHEN M D. Mechanism of sulfate attack: A fresh look. Part 2. Proposed mechanism [J]. Cement and Concrete Research, 2003, 33(2): 341-346.
[7] CAO H T, BUCEA L, RAY A, YOZGHATLIAN S. The effect of cement composition and pH of environment on sulfate resistance of Portland cements and blended cements [J]. Cement and Concrete Composites, 1997, 19(2): 161-171.
[8] FAMY C, TAYLOR H F W. Ettringite in hydration of Portland cement concrete and its occurrence in mature concretes [J]. ACI Mater J, 2001, 98: 350-6.
[9] GONZ?LEZ M A, IRASSAR E F. Ettringite formation in low C3A Portland cement exposed to sodium sulfate solution [J]. Cement and Concrete Research, 1997, 27(7): 1061-1072
[10] CRAMMOND N. The occurrence of thaumasite in modern construction—A review [J]. Cement and Concrete Composites, 2002, 24(4): 393-402.
[11] Thaumasite Expert Group. The thaumasite form of sulfate attack: Risks, diagnosis, remedial works and guidance on new construction [R]. London: DETR, 1999.
[12] COLLETTA G, CRAMMOND N J, SWAMY R N, SHARP J H. The role of carbon dioxide in the formation of thaumasite [J]. Cement and Concrete Research , 2004, 34(9): 1599-1612.
[13] MA Bao-guo, GAO Xiao-jian, BYARS E A, ZHOU Qi-zhi. Thaumasite formation in a tunnel of Bapanxia Dam in Western China [J]. Cement and Concrete Research, 2006, 36(4): 716-722.
[14] IRASSAR E F, BONAVETTI V L, GONZA?LEZ M. Microstructural study of sulfate attack on ordinary and limestone Portland cements at ambient temperature [J]. Cement and Concrete Research, 2003, 31(1): 31-41.
[15] LIU Ya-hui, SHEN Chun-ni, FANG Xiang-wei, XU Shou-bin, ZHANG Wei. Effects of solution concentration and temperature on deterioration rate of concrete by sulfate attack [J]. Joural of Chongqi Architecture University, 2008, 30(1): 129-135. (in Chinese)
(Edited by PENG Chao-qun)
Foundation item: Project(2008G025-C) supported by the Ministry of Railway of China; Project(50708114) supported by the National Natural Science Foundation of China
Received date: 2010-03-22; Accepted date: 2010-06-18
Corresponding author: LONG Guang-cheng, Professor, PhD; Tel: +86-731-82656568; E-mail: scc2005@mail.csu.edu.cn
- Deterioration of concrete in railway tunnel suffering from sulfate attack
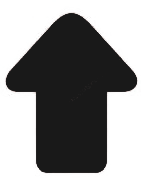