- Abstract:
- 1 Introduction▲
- 2 Materials and meth...▲
- 3 Results▲
- 4 Discussion▲
- 5 Conclusions▲
- References
- Figure
- Fig. 1 XRD patterns of TiNi substrates sintered at different temperatures:
- Fig. 2 Relative density of TiNi substrate
- Fig. 3 Surface morphology of TiNi alloys:
- Fig. 4 Surface morphology of TiNi alloys sintered at different temperatures:
- Fig. 5 XRD patterns of TiNi substrates with different raw materials:
- Fig. 6 Metallographs of as-sintered specimens after etching:
- Fig. 7 Surface morphology of TiNi alloys with different raw materials:
- Fig. 8 Effect of surface morphology on formation of apatite
- Fig. 9 Effect of pore size on formation of apatite
J. Cent. South Univ. (2016) 23: 1-9
DOI: 10.1007/s11771-016-3042-z
Calcium phosphate deposition on surface of porous and dense TiNi alloys in simulated body fluid
Liu Jue(刘珏), Liu Chao(刘超), Li Jing(李婧), Liu Min(刘敏), Ruan Jian-ming(阮建明)
State key laboratory of Powder Metallurgy, Central South University, Changsha 410083, China
Central South University Press and Springer-Verlag Berlin Heidelberg 2016
Abstract:
Porous and dense TiNi alloys were successfully fabricated by powder metallurgy (P/M) method, and to further improve their surface biocompatibility, surface modification techniques including grind using silicon-carbide (SiC) paper, acid etching and alkali treatment were employed to produce either irregularly rough surface or micro-porous surface roughness. X-ray diffractometry (XRD), scanning electron microscopy (SEM) and energy dispersive X-ray spectroscopy (EDX) attached to SEM were used to characterize surface structure and the Ca-P coatings. Effects of the above surface treatments on the surface morphology, apatite forming ability were systematically investigated. Results indicate that all the above surface treatments increase the apatite forming ability of TiNi alloys in varying degrees when soaked in simulated body fluid (SBF). More apatite coatings formed on TiNi samples sintered at 1050℃ and 1100℃ due to their high porosity and pure TiNi phase that is beneficial to heterogeneous nucleation. Furthermore, more uniform apatite was fabricated on the sample sintered from the mixture of Ni and Ti powders.
Key words:
calcium phosphate; TiNi alloy; surface treatment; microstructure; simulated body fluid (SBF);
1 Introduction
Titanium and titanium alloys are known to be used as biomaterials like medical devices due to their excellent properties [1-3]. Among them, TiNi alloy has been one of the materials of choice in total replacement of implants. Compared with traditional dense TiNi alloy, the porous TiNi alloy can not only keep the fine mechanical properties, but also show some unique advantages [4-5]. For example, the interconnected porous structure may facilitate the transportation of body fluids and further promote bone tissue ingrowth, which enables integration with the surrounding tissues more firmly, leading to the enhancement of interfacial stability. The implants of porous materials can also adjust modulus which is beneficial to reduce the extent of stress shielding [6-7]. Even though TiNi alloys share a relatively excellent bioactivity, they can not likely form a direct chemical bonding with bones, thus surface modification is critical to enhance bioactivity with Ca-P layers on porous TiNi alloy. During the last decades, many surface modification methods have been employed for the deposition of thin hydroxyapatite coatings, such as electrochemical deposition [8-9], plasma spraying [10-11], sputtering [12], dip coating [13] and electrophoretic deposition [14]. However, it is hard for these coating techniques to form apatite films on the inner surface of pores and almost all these methods are carried out at an elevated temperature at which post- sintering is required, which may cause deterioration of the metallic substrate [15].
So-called biomimetic technique, as a new way to form a thin hydroxyapatite film on the surface of substrates, is more and more widely used for its simple operation and less cost of money [16]. The Ca-P coatings are obtained by placing the substrates into SBF solution at 37 °C and pH 7.40, which is similar to human body condition. SBF, whose chemical components are similar to the inorganic mineral ions of human blood plasma and ion concentrations are also similar to those of human blood plasma, is a standard method by which the bioactivity of biomaterials is investigated [17-23].
As a big challenge to form biomimetic apatite on the surface of titanium and titanium alloys, different methods have been studied. For instance, Ugur and MUSTAFA [7] studied the effects of alkali and nitric acid surface treatment and acid etching on the Ca-P deposition of an open cell Ti6Al4V foam (60% porous and 300-500 μm in pore size) made for biomedical applications in a SBF solution for 14 d. And according to observations with scanning electron microscope (SEM), atomic force microscope (AFM), the coating layer thickness was about 3 μm in alkali treated foam samples while by nitric acid treatment, a relatively thin coating layer is 0.6 μm [7].
In another study, An et al [2] investigated acid etching prior to alkali- and heat-treatment of Ti to attain a surface with excellent affinity to bone. The surface examination results demonstrated that the roughness and wettability increased after pre-etching. Bone-like apatite was formed on pre-etched, alkali- and heat-treated surface. Osteoblasts showed better cellular compatibility on the pre-etched surface compared with the pure Ti surface or alkali- and heat-treated surface. Thus, acid etching prior to other pre-chemical treatments would be a method with bright future for improving the affinity of Ti to host bone tissues [2].
The outlined investigations and many other studies are mainly focused on Ca-P deposition on pure Ti and Ti6Al4V alloy with either comparably high porosity level or low porosity level [7, 24]. The present study is therefore conducted to investigate biomimetic Ca-P deposition on both dense and porous TiNi alloys with pre-chemical treatments.
2 Materials and methods
2.1 Sample preparation
Near-equiatomic TiNi samples were prepared by powder metallurgy method using pre-alloyed TiNi powders and the mixture of Ni and Ti powders. The average particle sizes of Ni and Ti powders were approximately 15.32 μm and 16.42 μm, respectively. The average particle size of pre-alloyed TiNi powders was 20.12 μm. The mixture of Ni and Ti powders was compacted under uniaxial pressure to form a green compact and then sintered at different temperatures (950, 1000, 1050, 1100 °C) for 2 h to obtain porous TiNi samples. Meanwhile, both the mixture of Ti and Ni powders and pre-alloyed TiNi powders were sintered by vacuum hot pressing sintering at 1100 °C and 25 Mpa to get dense TiNi samples. In order to note briefly, porous TiNi alloys are denoted as P950, P1000, P1050, P1100 according to different sintering temperatures. Dense TiNi alloys are denoted as D1 (the mixture of Ti and Ni powders) and D2 (pre-alloyed TiNi powders) according to different kinds of powder.
Those as-sintered substrates were further ground using silicon-carbide (SiC) paper of 240, 400, 800 and 1200 grit, respectively. After grinding the discs were cleaned in an ultrasonic bath for 30 min with acetone, ethanol, finally with distilled water, for removing the contaminated superficial layer.
2.2 Pre-chemical treatment
The cleaned samples were submitted to chemical treatment with 98% H2SO4 at room temperature for 30 min to remove the surface oxide layer. Then, the specimens were soaked in NaOH at the concentration of 5 mol/L, and temperature 60 °C for 24 h. Following the treatment, the samples were cleaned ultrasonically with distilled water for 30 min and dried at 40 °C in an electric oven overnight.
2.3 Preparation of SBF solution
in vitro bioactivity of biocomposites was investigated by SBF immersion. SBF, whose chemical components are similar to the inorganic mineral ions of human blood plasma and ion concentrations are also similar to those of human blood plasma, was prepared. At present, SBF is a standard method by which the bioactivity of biomaterials is investigated [17-18].
Since SBF is supersaturated with respect to apatite, an inappropriate preparation method may cause precipitation of apatite in the solution. reagents sodium chloride (NaCl, 8.035 g), sodium hydrogen carbonate (NaHCO3, 0.355 g), potassium chloride (KCl, 0.225 g), dipotassium hydrogen phosphate trihydrate (K2HPO4·3H2O, 0.231 g), magnesium chloride hexahydrate (MgCl2·6H2O, 0.311 g), calcium chloride dehydrate (CaCl2, 0.292 g), and sodium sulphate (Na2SO4, 0.072 g) in distilled water were dissolved at (36.5±1.5) °C under stirring. Then, pH was adjusted at 7.40 with tris-(hydroxymethyl)-aminomethane ((CH2OH)3CNH3) and hydrochloric acid (HCl) at 36.5 °C [17-18].
2.4 In vitro test
In vitro study was carried out by immersing the samples into SBF solution(placed in 100 ml conical flask) at 37 °C for 10 d and the SBF solution was refreshed every 2 d. After 10 d, samples were taken out and cleaned with distilled water, then dried in a drying oven at 40 °C for further characterization.
2.5 Characterization
The crystal structures of the phase of untreated and treated specimens before and after SBF immersion were determined by X-ray diffraction analysis(D/Max-2550, Japan) using Cu Kα (λ=1.5404 for 8.05 keV). A NOVATM Nano 230 scanning electron microscope (SEM) with an energy dispersive X-ray (EDX) analyzer was used for morphology analyses.
3 Results
XRD patterns of TiNi alloys sintered at different temperatures are shown in Fig. 1(a). When the sintering temperature is 950 °C, a lot of Ni and some secondary phase like Ti2Ni and TiNi3 are produced. When sintering temperature increases to 1000 °C, a little Ti2Ni and TiNi3 but no Ni exists. It shows that reaction between Ni and Ti is complete, but the interdiffusion is not sufficient, thus leading to composition segregation. At 1050 °C, no TiNi3 can be seen and just Ti2Ni phase remains. Moreover, the peaks of TiNi (austenite phase) and TiNi (martensite phase) can be seen from the XRD patterns in sample sintered at 1100 °C.
Fig. 1 XRD patterns of TiNi substrates sintered at different temperatures:
As Fig. 2 shows, with the increasing of sintering temperature, the relative density increases from 57% to 62%, that is to say, the porosity changes from 38% to 43%.
Fig. 2 Relative density of TiNi substrate
XRD profiles in Fig. 1(b) prove the formation of little sodium titanate, whose peaks are very weak. Comparing Fig. 1(b) with Fig. 1(a), the decreased peak intensity of TiNi at about 2θ=42° suggests that some amorphous phases may form during the pre-chemical treatments. Many researchers have obtained sodium titanate by chemical treatment, which is effective to improve bioactivity of species by inducing a bonelike apatite layer [6-7, 15, 25-26]. However, there is a little NaOH remaining on the sample P1000 during the cleaning process, which would not affect the results obtained. Figure 3(a) shows the morphology of the porous TiNi alloy. The porous TiNi alloy exhibited a three- dimensional interconnected structure. The pore size (average pore size 250 μm) and structure of the porous TiNi alloy closely match those of human bone. Surface morphologies of TiNi samples with different sintering temperatures change significantly after alkali treatment. Samples P950 and P1000 exhibit clear grain boundaries on the rough surface (Fig. 3(b), Fig. 3(c)); P1050 shows a pore-like structure on the surface (Fig. 3(d)); P1100 grows rough around the pores (Fig. 3(e)). EDX spectra (not shown) also show that elements like Ti, O, Ni, Na exist on the substrates after pre-chemical treatments, and the mole ratio of Na to Ti ranges from 0.22 to 0.29, also revealing the formation of sodium titanate.
XRD patterns of substrates after 10 d SBF immersion can be seen from Fig. 1(c). The peaks accord with the stardard PDF NO.09-0432, indicating the formation of hydroxyapatite phase. However, the hydroxyapatite shows an incomplete crystallization as peaks are weak and some kind of broadening [3]. According to XRD patterns obtained, there is no obvious apatite peaks in sample P1000; however, SEM observation shows that thin but dense apatite is formed on the surface of P1000 after 10 d [27]. Compared with the XRD patterns of the four TiNi alloys, the peaks of hydroapatite of sample P1050 and P1100 are relatively sharp and peak values are also higher. Therefore, the best deposition effect of HA could be got at 1050 °C and 1100 °C. At the same time, SEM micrographs shown in Fig. 4 demonstrate that the Ca-P layer formed from SBF solution consists of white particles with loose structure. At high magnification (×20000), the Ca-P film is composed of globules of 1-5 μm [1]. However, bubbles and fractures occur within the coatings formed and spherical particles in submicron size are attached to the surface of TiNi samples. In comparison with those SEM images of Ca-P coatings, deposition layers of P1050 and P1100 are thicker and white spherical particles are more and bigger. Further, Ca-P layer, clean and bright with no impurities, grows well near the pores. Moreover, from EDX result (not shown), thin films formed on the four substrates all have a composition with a Ca-to-P mole ratios less than 1.67 with a small amounts of Na, similar to mineral [28-29].
Fig. 3 Surface morphology of TiNi alloys:
With producing equiatomic TiNi alloys from the mixture of elemental Ti and Ni powders (sample D1) and pre-alloyed TiNi powders (sample D2), the average density of sample D1 is 6.323 g/cm3 and that of D2 is 6.427 g/cm3 (theoretical density of dense TiNi is 6.45 g/cm3), thus their relative densities are 98.03% and 99.64%, respectively. the XRD patterns of as-sintered samples show (Fig. 5(a)) that the two kinds of TiNi substrates exhibit obvious diffraction peaks at 2θ=42.48°,61.64°, 77.73°, which agree well with TiNi. Moreover, small amount of Ti2Ni (PDF#18-0898) can be found in D1 from XRD result. Figure 6 shows metallographs of D1 and D2. Grains of D1 are fine, dense and grainy in shape; however, D2 is obvious and grain size varies within the range of dozens of microns.
Fig. 4 Surface morphology of TiNi alloys sintered at different temperatures:
Two kinds of TiNi samples after grinding, acid etching, alkali treatment are assessed by XRD. Figure 5(b) shows that NaTi2O4 (PDF# 45-0752) diffraction peaks can be found in either D1 or D2. Yet, the NaTi2O4 diffraction peaks are weak and some even not obvious, especially from 10° to 38°. It shows the low degree of crystallinity of sodium titanate, or even sodium titanate may not exist as crystals but gel. Different compositions of TiNi alloys after chemical surface treatments are also characterized using SEM. In Figs. 7(a) and (b), D1 exhibits a rough surface with tiny pores which share a dispersive and uniform distribution. A small amount of pores exist on the surface of sample D1 and its porosity is also higher than that of D2. Surface of D2 is seriously damaged, with gully regions and holes distributing in length and breadth. Alkali treatment, however, results in relatively rough surface, contributing to the formation of bioactive thin Ca-P film.
Substrates after chemical treatments are soaked in SBF at 37 °C for 10 d, and evaluated by XRD. Figure 5(c) shows that intensities of diffraction peaks of Ca-P layer are weak and not so obvious. Small amount of submicro- to-micro sized particles are formed on the surface of D1. Due to distributing inhomogeneously, each of the particles exists alone with no connection each other. Moreover, a thin but obvious film attaches to substrate whose Ca-to-P ratio is 1.47, similar to 1.67 of hydroxyapatite (Fig. 7(c)). And the thin particles with spatial structure in Fig. 7(d) range from submicro to micro. According to EDX result (not shown), the white small particles formed on D2 could be related to nonstoichiometric hydroxyl- carbonate apatite [27, 30-31].
4 Discussion
Previous researches indicated that samples with higher surface roughness are regarded to strengthen the cell attachment to the implant surface, enhancing bioactivity between substrates and host tissues. Therefore, in order to obtain bioactive surface of TiNi alloys, acid etching and alkali treatment were conducted [2, 19-21, 32]. Surface impurities are removed by sandblasting, by which surface roughness efficiently increases. Acid etching with sulfuric acid (H2SO4) is expected to further remove the natural oxide layer on surface, speeding up the subsequent reactions [7, 33]. Corrosion rate of TiNi alloys increases with the acid concentration, so 98% concentrated H2SO4 at room temperature for 30 min was chosen. Alkali treatment, however, results in a relatively rough surface and sodium titanate is beneficial to the formation of Ca-P coatings [7, 33].
Fig. 5 XRD patterns of TiNi substrates with different raw materials:
Homogeneous but loose Ca-P coatings are deposited on TiNi substrates after soaking in SBF for 10 d. No matter porous or dense TiNi alloys or other titanium alloys, the formation mechanism of Ca-P coatings is the same [1-3, 6, 9, 16, 18, 28-29]. Sodium titanate formed during alkali treatment is found to be hydrolyzed. Na+ ions releasing to SBF solution are substituted by H3O+ ions, and Ti—OH groups form on surface, leading to pH increasing. Negative charged Ti—OH groups would absorb positive charged Ca2+ groups by electrostatic force at first, and Ca2+ groups attract negative charged Then Ca2+ and
ions are attracted to the surface of substrates; supersaturation of Ca and P increases due to pH increase, thus further promoting deposition of Ca2+ and
The enrichment of both Ca2+ and
ions increases the local supersaturation degree with respect to hydroxyapatite and its ionic activity product according to the following equilibrium in the SBF:
=Ca10(PO4)6(OH)2+6H2O
Fig. 6 Metallographs of as-sintered specimens after etching:
The increased ionic activity product accelerates apatite nucleation on TiNi substrates. During the process, the apatite Ca-P particles are mainly deposited on the surface of the TiNi sample and very little precipitation occurs on the bottom of the container due to the competition between heterogeneous nucleation around the surface and homogeneous nucleation in the solution [3, 28]. Formation of hydroxyapatite, consisting of nucleating, growing and aggregation of new phase, is exactly a complete crystallization process. In SBF solution, Ca2+ and ions are attracted to TiNi surface by electrostatic interaction and react each other, thus a solid-liquid interface is formed due to precipitation of new phase. The free energy for nucleation, ΔG, is sharply reduced during the formation of new phase; however, interfacial energy, △GS, increases greatly. Once the nuclei seeds are formed, they can spontaneously grow by consuming Ca and phosphate ions from SBF [3].
Fig. 7 Surface morphology of TiNi alloys with different raw materials:
Surface morphology demonstrates a very significant effect on formation of Ca-P coatings from SBF solution. The Ca-P nuclei seeds are firstly deposited on TiNi substrates and then expanded by continuously consuming Ca and P ions from the solution [29, 34]. The heterogeneous nucleation of Ca-P seeds easily happens on rough surface. Figure 8 shows that the rough surface obtained in grinding by sandpaper is macroscopically covered with almost parallel grooves and ridges. acid etching and alkali treatments further increase surface roughness and deepen grooves. These grooves and ridges, which provide nucleating sites, can keep a relatively high ions concentration and reach nucleating threshold fast. Therefore, local concentrations of Ca2+ and ions on substrates are important for nucleation, contributing to structure and composition of crystals changing in the nucleation process. Moreover, nucleating at grain boundaries can reduce interfacial energy, which leads nucleation energy to decreasing, thus driving crystals to grow [3].
Fig. 8 Effect of surface morphology on formation of apatite
As we know, bioactivity has a close relationship with porosity and pore size of substrates. The higher the porosity is, the more the surface area is, thus leading to more particles to form. Liang et al [16] declared that if the pore size on the surface is big, the solute can reach the bottom of the pores easily, so apatite nuclei can grow because they can absorb Ca2+ and ions continuously. Instead, if the pore size on the surface is too small, the bottom is shielded by low solute concentration region, which may not benefit to the nucleation and growth of apatite [16]. Cells can grow into pores whose size is 10 μm, pore size of 10-50 μm is benefit to ingrowth of fibrous tissues, and 100-500 μm can also be beneficial to newly-born bone tissue. However, superficial pores whose pore size is less than 100 μm can not provide a favorable condition to the nucleation (Fig. 9). Figure 2 shows that porosity of TiNi samples sintered from 950 °C to 1100 °C varies from 38% to 43%, which belong to porous materials. In comparison with dense TiNi alloy, the porous one sharing higher surface areas has a worse corrosion resistance, thus leading to better corrosion effects after chemical treatments with H2SO4 and NaOH. From the results obtained, denser and thicker apatite formed on samples P1050 and P1100. At a relatively close porosity level, other influencing factors become particularly obvious. XRD patterns in Fig. 1(a) show that reactions between Ni and Ti are completed and no TiNi3 can be seen just Ti2Ni phase remains in P1050. Moreover, only TiNi (austenite phase) and TiNi (martensite phase) can be found in P1100. More pure and beneficial TiNi phase exhibits a better bioactivity, which leads to thicker apatite forming on surfaces on P1050 and P1100, undoubtedly [4-5].
Fig. 9 Effect of pore size on formation of apatite
Decreasing nucleation energy, at areas with high curvature like the edge of holes and tips, will stimulate nucleation. Sample D1 is produced by the chemical reaction of mixed Ni and Ti powders, contributing to sharping of TiNi diffraction peaks. Sample D2 is processed by solid phase diffusion between elements Ti and Ni, leading to diffraction peaks widening due to severe lattice distortion and lattice constant changing. And the diffusion rate of Ni is faster than that of Ti, so the content of Ti could be higher in some area, generating little Ti2Ni secondary phase during cooling process (TiNi→TiNi+Ti2Ni). At an equal energy state, most energy in D2 applies to diffusion between particles and grain growth. Furthermore, composition of this kind of sample is comparatively even, thus leading to a relatively low resistance for grain growth. However, energy of D1 not only applies to the diffusion of particles and grain growth, but also to the reaction between Ni and Ti powders. Furthermore, Ti powder, showing a strong affinity to impurity elements which is enriched in the grain boundaries, can prevent further growth of grains and make grains fine and dense. From the metallographs of sintered specimens after etching in Fig. 6, grains of sample D1 are fine and uniform, which may contribute to the increased number of heterogeneous nucleation sites [7]. Hence, loose but uniform Ca-P coatings form better on sample D1 than D2.
5 Conclusions
Using pre-alloyed TiNi powders and the mixture of elemental Ni and Ti powders, porous and dense substrates were prepared by powder metallurgy process. All of samples were subjected to surface modification techniques including grind using silicon-carbide (SiC) paper, acid etching and alkali treatment and soaked in SBF for 10 d to form apatite coatings on surface of different samples. Dense and thick Ca-P coatings formed on the TiNi alloys sintered at 1050 °C and 1100 °C due to their relatively high porosity and pure TiNi phase that is beneficial to heterogeneous nucleation. And more uniform Ca-P film was fabricated on sample produced by the mixture of Ni and Ti powders.
References
[1] Barrere F, van Blitterswijk C A, de Groot K, Layrolle P. Nucleation of biomimetic Ca-P coatings on Ti6Al4V from a SBF×5 solution: influence of magnesium [J]. Biomaterials, 2002, 23: 2211-2220.
[2] An S H, Matsumoto T, Miyajima H, Sasaki J I, Narayanan R, Kim K H. Surface characterization of alkali- and heat-treated Ti with or without prior acid etching [J]. Appl Surf Sci, 2012, 258: 4377-4382.
[3] Chen Xiao-bo, Li Yun-cang, Hodgson P D, Wen Cui-e. Microstructures and bond strengths of the calcium phosphate coatings formed on titanium from different simulated body fluids [J]. Mater Sci Eng C, 2009, 29: 165-171.
[4] Zhang J X, Guan R F, Zhang X P. Synthesis and characterization of sol-gel hydroxyapatite coatings deposited on porous TiNi alloys [J]. J Alloy Compd, 2011, 509: 4643-4648.
[5] Zheng C Y, Nie F L, Zheng Y F, Cheng Y, Wei S C, Valiev R Z. Enhanced in vitro biocompatibility of ultrafine-grained biomedical TiNi alloy with microporous surface [J]. Appl Surf Sci, 2011, 257: 9086-9093.
[6] Ribeiro A A, Balestra R M, Rocha M N, Peripolli S B, Andrade M C, Pereira L C, Oliveira M V. Dense and porous titanium substrates with a biomimetic calcium phosphate coating [J]. Appl Surf Sci, 2013, 265: 250-256.
[7] Ugur T, Mustafa G. The effect of surface treatment on CaP deposition of Ti6Al4V open cell foams in SBF solution [J]. Ceram Int, 2010, 36: 1805-1816.
[8] Zhang Qi-yi, Leng Yang, Xin Ren-long. A comparative study of electrochemical deposition and biomimetic deposition of calcium phosphate on porous titanium [J]. Biomaterials, 2005, 26: 2857-2865.
[9] Monma H. Electrolytic depositions of calcium phosphates on substrate [J]. J Mater Sci, 1994, 29: 949-953.
[10] Filaggi M J, Coombs N A, Pilliar R M. Characterization of the interface in the plasma-sprayed HA coating/Ti-6Al-4V implant system [J]. J Biomed Mater Res, 1991, 25: 1211-1219.
[11] Hayashi K, Mashima T, Uenoyama K. The effect of hydroxyapatite coating on bony ingrowth into grooved titanium implants [J]. Biomaterials, 1999, 20: 111-119.
[12] Lacefield W R. Hydroxyapatite coatings [J]. Ann Biomed Mater Res, 1988, 523: 73-80.
[13] Boulton L M, Gregson P J, Tuke M, Baldwin T. Adhesively bonded hydroxyapatite coating [J]. Mater Lett, 1991, 12: 1-6.
[14] Wei M, Ruys A J, Milthorpe B K, Sorrell C C. Solution ripening of hydroxyapatite nanoparticles: effects on electrophoretic deposition [J]. J Biomed Mater Res, 1999, 45: 11-19.
[15] Wei M, Kim H M, Kokubo T, Evans J H. Optimising the bioactivity of alkaline-treated titanium alloy [J]. Mater Sci Eng C, 2002, 20: 125-134.
[16] Liang Fang-hui, Zhou Lian, Wang Ke-guang. Apatite formation on porous titanium by alkali and heat-treatment [J]. Surf Coat Tech, 2003, 165: 133-139.
[17] Kokubo T, Takadama H. How useful is SBF in predicting in vivo bone bioactivity [J]. Biomaterials, 2006, 27: 2907-2915.
[18] Kokubo Tadashi. Design of bioactive bone substitutes based on biomineralization process [J]. Mater Sci Eng C, 2005, 25: 97-104.
[19] Kim H M, Miyaji F, Kokubo T, Nakamura T. Bonding strength of bonelike apatite layer to Ti metal substrate [J]. J Biomed Mater Res, 1997, 38: 121-127.
[20] Kim H M, Miyaji F, Kokubo T, Nishiguchi S, Nakamura T. Graded surface structure of bioactive titanium prepared by chemical treatment [J]. J Biomed Mater Res, 1999, 45: 100-107.
[21] Wen H B, Liu Q, de Wijin J R, de Groot K, Cui F Z. Preparation of bioactive microporous titanium surface by a new two-step chemical treatment [J]. J Mater Sci Mater Med, 1998, 9: 121-128.
[22] Li P, Ohtsuki C, Kokubo T, Nakanishi K, Soga N, de Groot K. The role of hydrated silica, titania, and alumina in inducing apatite on implants [J]. J Biomed Mater Res, 1994, 28: 7-15.
[23] Kokubo T, Miyaji F, Kim H M, Nakamura T. Spontaneous apatite formation on chemically treated titanium metals [J]. J Am Ceram Soc, 1996, 79: 1127-1129.
[24] Adriana B, Elisa B, Barbara B, Alessandro F, Silvia P, Francesco S, Luigina S. Nanocrystalline hydroxyapatite coatings on titanium: a new fast biomimetic method [J]. Biomaterials, 2005, 26: 4085-4089.
[25] Lee B H, Kim Y D, Shin J H, Lee K H. Surface modification by alkali and heat treatments in titanium alloys [J]. J Biomed Mater Res, 2002, 61: 466-473.
[26] Turkan U, Guden M. The effect of nitric acid surface treatment on CaP deposition of Ti6Al4V open-cell foams in SBF solution [J]. Surf Coat Tech, 2010, 205: 1904-1916.
[27] Sepahvandi A, Moztarzadeh F, Mozafari M, Ghaffari M, Raee N. Photoluminescence in the characterization and early detection of biomimetic bone-like apatite formation on the surface of alkaline-treated titanium implant: State of the art [J]. Colloid Surface B, 2011, 86: 390-396.
[28] Kokubo T, Matsushita T, Takadama H, Kizuki T. Development of bioactive materials based on surface chemistry [J]. J Eur Ceram Soc, 2009, 29: 1267-1274.
[29] Takadama H, Kim H M, Kokubo T, Nakamura T. TEM-EDX study of mechanism of bonelike apatite formation on bioactive titanium metal in simulated body fluid [J]. J Biomed Mater Res, 2001, 57: 441-448.
[30] Mozafari M, Moztarzadeh F. Controllable synthesis, characterization and optical properties of colloidal PbS/gelatin core-shell nanocrystals [J]. J Colloid Interf Sci, 2010, 351: 442-448.
[31] Mozafari M, Moztarzadeh F, Tahriri M. Green synthesis and characterization of spherical PbS luminescent nanocrystals via wet-chemical technique [J]. Adv Appl Ceram, 2011, 110: 30-34.
[32] Kim H M, Miyaji F, Kokubo T, Nakamura T. Apatite forming ability of alkali-treated Ti metal in body environment [J]. J Ceram Soc Jpn, 1997, 105: 111-116.
[33] Lu X, Wang Y B, Yang X D, Zhang Q Y, Zhao Z F, Weng L T, Leng Y. Spectroscopic analysis of titanium surface functional groups under various surface modification and their behaviors in vitro and in vivo [J]. J Biomed Mater Res A, 2008, 84A: 523-534.
[34] Kim H M, Miyaji F, Kokubo T. Effect of heat treatment on apatite-forming ability of Ti metal induced by alkali treatment [J]. J Mater Sci Mater Med, 1997, 8: 341-347.
(Edited by YANG Hua)
Foundation item: Project(51274247) supported by the National Natural Science Foundation of China; Project(2014zzts177) support by the Fundamental Research Funds for the Central Universities, China
Received date: 2014-11-24; Accepted date: 2015-07-22
Corresponding author: Ruan Jian-ming, professor; Tel/Fax: +86-731-88836827; E-mail: jianming@csu.edu.cn
Abstract: Porous and dense TiNi alloys were successfully fabricated by powder metallurgy (P/M) method, and to further improve their surface biocompatibility, surface modification techniques including grind using silicon-carbide (SiC) paper, acid etching and alkali treatment were employed to produce either irregularly rough surface or micro-porous surface roughness. X-ray diffractometry (XRD), scanning electron microscopy (SEM) and energy dispersive X-ray spectroscopy (EDX) attached to SEM were used to characterize surface structure and the Ca-P coatings. Effects of the above surface treatments on the surface morphology, apatite forming ability were systematically investigated. Results indicate that all the above surface treatments increase the apatite forming ability of TiNi alloys in varying degrees when soaked in simulated body fluid (SBF). More apatite coatings formed on TiNi samples sintered at 1050℃ and 1100℃ due to their high porosity and pure TiNi phase that is beneficial to heterogeneous nucleation. Furthermore, more uniform apatite was fabricated on the sample sintered from the mixture of Ni and Ti powders.
- Calcium phosphate deposition on surface of porous and dense TiNi alloys in simulated body fluid
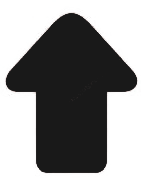