- Abstract:
- 1 Introduction▲
- 2 Experimental▲
- 3 Results and discussion▲
- 4 Conclusions▲
- References
- Figure
- Fig.1 X-ray diffraction patterns (a) and TG curves (b) of raw CS(C1), AC/n-CdS/CS (C2) and n-CdS/CS (C3)
- Fig.2 Comparison of only photolysis, adsorption in dark and visible light photocatalysis of MO (Catalyst amount = 1.0 g/L, C0 = 10 mg/L, pH = 6.0)
- Fig.3 UV-Vis spectra changes of MO for adsorption in dark (a) and photocatalysis under visible light irradiation (b) (Catalyst amount=1.0 g/L, C0=10 mg/L, pH=6.0)
- Fig.4 Influence of catalyst amount on decolorization efficiency of MO (C0=10 mg/L, pH=6.0)
- Fig.5 Relationship between different initial MO concentrations and irradiation time (a) and fitted curve between 1/r0 and 1/C0 at different initial concentrations (b) (Catalyst amount=1.0 g/L, pH=6.0)
- Fig.6 Influence of initial pH on decolorization efficiency of MO (Catalyst amount=1.0 g/L, C0=10 mg/L)
- Fig.7 Reuse of catalyst on MO decolorization efficiency (Catalyst amount = 1.0 g/L, C0 =10 mg/L, pH = 6.0)
J. Cent. South Univ. Technol. (2010) 17: 1223-1229
DOI: 10.1007/s11771-010-0623-0
Synergy of adsorption and visible light photocatalysis to decolor methyl orange by activated carbon/nanosized CdS/chitosan composite
JIANG Ru(蒋茹)1, 2, ZHU Hua-yue(朱华跃)2, 3, ZENG Guang-ming(曾光明)1,
XIAO Ling(肖玲)3, GUAN Yu-jiang(管玉江)2
1. College of Environmental Science and Engineering, Hunan University, Changsha 410082, China;
2. Department of Environmental Engineering, Taizhou University, Linhai 317000, China;
3. College of Resource and Environmental Science, Wuhan University, Wuhan 430072, China
? Central South University Press and Springer-Verlag Berlin Heidelberg 2010
Abstract:
Activated carbon/nanosized CdS/chitosan (AC/n-CdS/CS) composites as adsorbent and photoactive catalyst were prepared under low temperature (≤60 ℃) and ambient pressure. Methyl orange (MO) was chosen as a model pollutant to evaluate synergistic effect of adsorption and photocatalytic decolorization by this innovative photocatalyst under visible light irradiation. Effects of various parameters such as catalyst amount, initial MO concentration, solution pH and reuse of catalyst on the decolorization of MO were investigated to optimize operational conditions. The decolorization of MO catalyzed by AC/n-CdS/CS fits the Langmuir- Hinshelwood kinetics model, and a surface reaction, where the dyes are absorbed, is the controlling step of the process. Decolorization efficiency of MO is improved with the increase in catalyst amount within a certain range. The photodecolorization of MO is more efficient in acidic media than alkaline media. The decolorization efficiency of MO is still higher than 84% after five cycles and 60 min under visible light irradiation, which confirms the reusability of AC/n-CdS/CS composite catalyst.
Key words:
1 Introduction
Heterogeneous photocatalysis has recently emerged as an effective and promising advanced oxidation technology to treat wastewater [1-2]. The key technology involving in heterogeneous photocatalysis is the choice and preparation of cost-effective and feasible semiconductor materials. Among several usual semiconductors, titanium dioxide (TiO2) has been widely studied due to its many merits, such as high efficiency, chemical stabilization and low cost [2-4]. However, practical application of TiO2 is limited by two main factors: (1) high rate of electron/hole pairs recombination reduces quantum yield of TiO2 during photocatalytic process; (2) the need of using an ultraviolet wavelength (<387 nm) as excitation source can only capture less than 5% of the solar energy arrived at the Earth’s surface due to its wide band gap of 3.2 eV [3, 5]. For the sake of effective use of sunlight, cadmium sulfide (CdS), with narrow band gap of 2.5 eV, has attracted more and more attention in recent years [6-12]. However, CdS nanoparticles are easy to aggregate in aqueous solution during preparation process. Researchers have explored some methods to control their size and avoid aggregation, such as by producing these particles in micellar media [6], capping the surface of particles [7-8] and matrices [9].
Chitosan (CS), (1,4)-2-amino-2-deoxy-β-D-glucosa- mine, is a natural alkaline hydrophilic, nontoxic and biocompatible biopolymer obtained by the alkaline deacetylation of chitin [13]. Chitosan has good chelating ability with transition metal ions [14-15], which makes it possible for its metal ion complexes to be used as precursors to synthesize metal nanoparticle–chitosan composite [16-18]. In addition, amino (—NH2) and hydroxyl groups (—OH) on linear chitosan chains are good capping groups for preparing heterogeneous catalysts. Besides, the highly viscous nature of chitosan can also prevent nanoparticles from agglomeration during the growth. Additionally, chitosan is well-known excellent adsorbent for a number of organic dyes [19-20], of which the sensitization can further increase the photocatalytic activity of composite catalysts. In our previous researches, nanosized CdS/chitosan (n-CdS/CS) was prepared at low temperature (≤ 60 ℃) and under ambient pressure. It was proved that n-CdS/CS had higher decolorization of dyes [21-22]. However, the use of cross-linking agent glutaraldehyde decreases the ability of adsorbing pollutants. Activated carbon (AC) has a large specific surface area and a well developed porous structure, resulting in an attractive force toward organic dye molecules and heavy metals. As a result, AC is widely used as a support of catalyst in gas and water remediation [23-30]. The introduction of both chitosan and AC to synthesize activated carbon/nanosized CdS/chitosan (AC/n-CdS/CS) composite is expected to produce synergistic effect of adsorption and photocatalysis.
In this work, preparation, characterization and adsorption-photocatalysis of AC/n-CdS/CS composite were reported. Methyl orange (MO) was chosen as a model compound to evaluate the decolorization ability of AC/n-CdS/CS under visible light irradiation. For practical use of AC/n-CdS/CS in dye wastewater treatment, there is a need to determine optimal conditions of experimental parameters, such as catalyst amount, dye initial concentration, solution pH and recycle of catalyst for effective decolorization of MO.
2 Experimental
2.1 Materials
CS was purchased from Zhejiang Yuhuan Jinke Biochemisty Industry Co., LTD (Taizhou, China) and used without further purification. The degree of deacetylation was 91.7% and its average relative molecular mass was 2.1×104. Commercially available AC (Tianjin, China) was used, which was produced by the vapor activation of coconut shell and had the grain size about 0.12 cm. The dye, methyl orange (C.I.13025, C14H14O3N3SNa), was purchased from Zhejiang Yongjia Fine Chemical Factory (Wenzhou, China) and used as received. Other chemicals used in the experiments, cadmium chloride, thiocarbamide ((NH2)2CS), and glutaraldehyde, were of analytical grade. All solutions were prepared in double distilled water.
2.2 Preparation of AC/n-CdS/CS composite catalyst
The AC/n-CdS/CS composites were prepared by simulating bio-mineralization method modified from previous literatures [21-22]. At room temperature, 1.5 g CS was dissolved in 100 mL 0.2 mol/L nitric acid and stirred for 2 h. Subsequently, 2 g AC was added into the solution and stirred for 30 min to disperse AC uniformly. Then, 0.913 4 g CdCl2 dissolved in 20 mL water was added into CS colloidal solution and the dispersion mixture was continuously stirred for 4 h to facilitate a chelating balance. After the chelating equimolar amounts thiocarbamide aqueous solution was dropped slowly into the dispersion mixture in a water bath at 60 ℃. The mixture was immersed into 100 mL 0.25% glutaraldehyde solution and stirred for 30 min. Finally, sodium hydroxide (200 mL, 0.1 mol/L) aqueous solution was introduced to precipitate composite particles. The brown products (AC/n-CdS/CS) were filtered and washed with distilled water and absolute ethyl alcohol for 3-4 times, respectively, and dried at 60 ℃ under atmospheric condition.
2.3 Characterization of AC/n-CdS/CS composite
For crystal structure analysis of the prepared samples, X-ray powder diffraction (XRD) analysis was carried out on a Bruker D8 ADVANCE (German) X-ray diffractometer with Cu Kα radiation (λ=0.154 06 nm, U= 40 kV, I=50 mA) at a scan rate of 0.02 (?)/s in the 2θ range from 5? to 70?. Thermogravimetry (TG) was performed using a Setsys 16 TG/DTA/DSC (Setaram, France) under a nitrogen atmosphere of 0.15 MPa from 25 to 800 ℃ with heating rate of 2 ℃/min. Sample amount was about 20 mg.
2.4 Photocatalytic reaction
A 300 W xenon lamp (PLS-SXE300, Beijing Trusttech Co. Ltd, China) loaded with a UV-cut filter, which basically emits at 400-780 nm, was used as visible light source. The light intensity of visible light was kept approximately constant at 2 W/cm2 and 10 cm away from the light source. Photocatalytic oxidation experiments were carried out using a cylindrical organic glass reactor (80 mm in diameter and 110 mm in depth) in the open-air condition. The suspensions with catalysts were magnetically stirred using a thermostat magnetic stirrer (model 85-2, Gongyi Yuhua Instrument Co. LTD, China) and continuously aerated by air pump to provide oxygen for the mixing of reaction solution. 5 mL of the suspension was withdrawn from the reactor vessel periodically and centrifuged at 10 000 r/min in order to determine the change of MO concentration. Then, the supernate was analyzed by Cary 50 UV-Vis spectro- photometer (Varian, USA) controlled by a Lenovo PC. All of the experiments were conducted at constant temperature of 25 ℃.
In order to determine the optimal catalyst amount on the MO decolorization, a series of experiments were performed varying from 0.2 to 1.5 g/L, at 10 mg/L dye concentration and pH 6.0. A series of MO solutions over the concentration range of about 5 to 40 mg/L were used to observe the effect of initial dye concentration on decolorization. The initial pH values investigated for the AC/n-CdS/CS photocatalytic decolorization were 2.0, 4.0, 6.0 and 8.0, respectively when MO concentration was 10 mg/L and catalyst amount was 1.0 g/L. The pH of solution was adjusted using 0.1 mol/L dilute sulfuric acid or 0.1 mol/L sodium hydroxide and monitored by a model 990 pH/conductivity meter (Jiangsu, China). The catalyst was recycled four times at 10 mg/L dye concentration in the presence of 1.0 g/L catalyst at pH 6.0. After each experiment, the catalyst was centrifuged for 15 min, washed and recycled.
The decolorization efficiency of MO is defined as follows:
×100% (1)
where η is decolorization efficiency of MO; C is the concentration of MO at reaction time t (mg/L); and C0 is the initial concentration of MO (mg/L).
Generally, the kinetic reaction mechanism of photocatalytic degradation conforms to the Langmuir- Hinshelwood (L-H) model [30-31], which is commonly expressed as the following equation:
(2)
where r0 is the initial photocatalytic decolorization efficiency (mg/(L?min)); k is the apparent reaction efficiency constant (mg/(L?min)); and KA is the adsorption equilibrium constant (L/mg).
Linearization of Eq.(2) gives the relationship:
(3)
3 Results and discussion
3.1 Characterization of AC/n-CdS/CS composite
3.1.1 X-ray diffraction analysis
For structural analysis, X-ray power diffraction analysis was employed. Typical XRD patterns of CS (C1), AC/n-CdS/CS (C2) and n-CdS/CS (C3) composite are shown in Fig.1(a). The diffraction spectrum of CS (C1) exhibits only two major crystalline peaks at 2θ of 11.8? and 20.9?, while there are six additional peaks in patterns of both AC/n-CdS/CS (C2) and n-CdS/CS (C3), corresponding to the (100), (002), (101), (110), (103) and (112) planes of the hexagonal phase of CdS phase (JCPDS 06-0314) [32]. This shows the successful formation of hexagonal phase of CdS on both AC/n-CdS/CS (C2) and n-CdS/CS (C3). On the other hand, the peaks on the pattern of AC/n-CdS/CS (C2) are broader compared with the pattern of n-CdS/CS (C3), indicating that CdS nanoparticles on AC/n-CdS/CS (C2) are smaller. The average crystalline size of CdS is estimated from the FWHM (full-width at half-maximum) of diffraction peaks using the Debye-Scherrer formula [12].
D=kl/(βcos θ) (4)
where D is the mean particle size (nm); λ is X-ray wavelength used in experiment; β is the half-width of the diffraction peak and can be measured from the XRD pattern; and θ is the corresponding diffraction angle. Here, 2θ values corresponding to (110) and (103) plane are selected. The average crystalline size of CdS nanoparticles in AC/n-CdS/CS is 20-25 nm.
Fig.1 X-ray diffraction patterns (a) and TG curves (b) of raw CS(C1), AC/n-CdS/CS (C2) and n-CdS/CS (C3)
3.1.2 Thermal analysis
TG curves of CS (C1), AC/n-CdS/CS (C2) and n-CdS/CS (C3) are shown in Fig.1(b). The TG curves show that three mass losses occur mainly at 25-800 ℃. The initial mass loss for three samples from 25 to 200 ℃ corresponds to the evaporation of physically adsorbed water molecules weakly bound to the materials [33]. The other two steps at 250-500 ℃ and 500- 800 ℃ are caused by the decomposition of residual organic group. The total mass loss at 800 ℃ is 72%, 58% and 36% for CS (C1), n-CdS/CS (C3) and AC/n- CdS/CS (C2), respectively. The total mass loss of AC/n-CdS/CS is less about 36% and 22% than that of CS and n-CdS/CS, respectively. At first, it can be attributed to the changes in the polymer natural order due to chelating process, leading to thermal stability [34]. Additionally, there is more inorganic compound in AC/n-CdS/CS than both pure CS and n-CdS/CS. As a result, AC/n-CdS/CS composites exhibit better thermal stability than both pure CS and n-CdS/CS.
3.2 Synergic effect of adsorption and photocatalytic activity
In order to investigate the adsorption and photocatalysis of AC/n-CdS/CS composites, decolorization experiments of MO by photocatalysis under artificial visible light irradiation and adsorption in dark were carried out. All results are shown in Fig.2. The blank experiment under visible light irradiation without catalysts indicates that only photolysis can be ignored as almost no decolorization occurred for 80 min in MO solution. It is well known that adsorption is very important to the decolorization of organic dye pollutants. For adsorption on the surface of composite catalyst in the absence of visible light irradiation, the decolorization efficiency of MO reaches 22.3% and 71.37% after 10 and 80 min of adsorption, respectively. This is due to the adsorption ability of AC/n-CdS/CS composite resulting from large surface area of AC and the residual —NH2 and —OH groups in crosslinked CS. While for visible light photocatalysis in the presence of AC/n-CdS/CS composite, the decolorization efficiency of MO increases by 30.8% and 22.1%, respectively, compared with the corresponding period of adsorption in dark. This shows that there exists simultaneously adsorption and photocatalysis on the surface of composite in the presence of visible light irradiation. The adsorption ability of AC/n-CdS/CS enhances the photocatalysis of MO, and the photocatalysis and adsorption produce synergistic effects for the dye decolorization.
Fig.2 Comparison of only photolysis, adsorption in dark and visible light photocatalysis of MO (Catalyst amount = 1.0 g/L, C0 = 10 mg/L, pH = 6.0)
To compare different decolorizations of MO using different treatment processes, representative UV-Vis spectra changes of the dye solution as a function of reaction time for adsorption in dark and photocatalysis under visible light irradiation were recorded. The results are depicted in Fig.3. As observed from these spectra, the primary absorption peaks of original dye solution are 271.0 and 464.9 nm in the range of 200-560 nm, which are attributed to benzene and azo linkage, respectively. During adsorption in dark (Fig.3(a)), as the reaction time increases, the intensities of two peaks decrease gradually. However, the situations of both peaks are not changed after 80 min treatment. The two absorbency peaks at 271.0 and 464.9 nm trail off evidently and shift to 278.0 and 452.8 nm after 10 min treatment, respectively. The ultraviolet peak and visible peak disappear entirely at 20 and 40 min (Fig.3(b)), respectively. These results indicate that the aromatic rings at 271.0 nm and azo bonds at 464.9 nm of MO are destroyed partly under visible light irradiation.
Fig.3 UV-Vis spectra changes of MO for adsorption in dark (a) and photocatalysis under visible light irradiation (b) (Catalyst amount=1.0 g/L, C0=10 mg/L, pH=6.0)
3.3 Effect of operational parameters on photocatalytic decolorization
3.3.1 Effect of catalyst amount
Catalyst amount is an important parameter of the photocatalytic process. From Fig.4, it is observed that as the catalyst amount increases from 0.2 to 1.0 g/L, the MO concentration in the solution decreases rapidly and the decolorization efficiency increases from 52.7% to 92.4% after 60 min. This is because when catalyst concentration increases, the number of photos absorbed and the number of dye molecules adsorbed are increased [30, 34]. While the catalyst amount is increased from 1.0 to 1.5 g/L, the decolorization efficiency increases to 93.5% at the end of the 60 min, showing no evident enhancement because of the screening effect of light. So, the following experiments are performed using 1.0 g/L catalyst amount.
Fig.4 Influence of catalyst amount on decolorization efficiency of MO (C0=10 mg/L, pH=6.0)
3.3.2 Effect of initial dye concentration and kinetics analysis
It is important to study the dependence of initial substrate concentration on decolorization kinetics of the pollutant both from mechanism and application points of view. The relationship between initial MO concentration and irradiation time catalyzed by AC/n-CdS/CS is plotted in Fig.5(a).
Based on the removal curves, the initial decolorization efficiencies r0 could be calculated. In this section, 1/r0 was plotted versus 1/C0 based on the data from Fig.5(a). As shown fitted in Fig.5(b), 1/r0 correlated to 1/C0 well, so the photocatalytic decolorization of MO catalyze by AC/n-CdS/CS fitted with the Langmuir- Hinshelwood (L-H) kinetics model. According to the Langmuir-Hinshelwood kinetics in Fig.5(b), straight line is obtained with a stope of 1/k and an intercept of 1/(kKA), from which k and KA are estimated to be 5.263 mg/(L?min) and 0.015 8 L/mg respectively. The regression line has a correlation coefficient of 0.999 4. The result of k>KA suggests that a surface reaction, where the dyes are absorbed, is the controlling step of the process [30].
3.3.3 Effect of solution pH
The solution pH plays an important role in the generation of ×OH radical [35] and thus is one of the important parameters to be studied. As shown in Fig.6, the decolorization efficiency of MO solution for pH of 2.0, 4.0, 6.0 and 8.0 is 96.1%, 80.2%, 52.9% and 18.2%, respectively, after 10 min of reaction. After 60 min of reaction, the decolorization efficiency is 97.2%, 96.1%, 92.4% and 88.1%, respectively. The results indicate that the photocatalytic decolorization of MO is more efficient in acidic media than alkaline media.
MO molecule with sulfuric group ionizes easily in
acidic media and becomes a soluble MO anion. The surface of CdS is positively charged once CdS is evoked by relevant light to create electrons, which are then scavenged by molecular oxygen O2 to yield the superoxide radical anion O2-· and hydrogen peroxide H2O2 in oxygen-equilibrated media [31]. At the same time, residual amino groups of CS in AC/n-CdS/CS are much more easily to be protonated at low pH and could form electrostatic attraction to adsorb a quantity of dye anions [36]. Therefore, in acidic solution, MO anions are easily adsorbed to AC/n-CdS/CS with positive surface charge, while MO anions are generally excluded away from surface of catalyst in alkaline media. MO anions could be oxidized more directly by hydroxyl radicals produced under visible light excitation in acidic media. That is why higher decolorization efficiencies are obtained in acidic media. The possible decolorization mechanism of MO on AC/n-CdS/CS composites might be expressed as follows [22]:
(1) The first step is mainly the adsorption of azo anions on CS:
R1—NH3++R2—SO3-=R1—NH3+·O3S—R2
where R1—NH3+ is the CS; and R2—SO3- is the dye anion.
(2) The second step is photocatalytic decolorization of MO on AC/n-CdS/CS:
CdS+hν?CdS(h+)+CdS(e-)
CdS(h+)+H2O?CdS+·OH+H+
·OH+RH?Degradation product
Fig.5 Relationship between different initial MO concentrations and irradiation time (a) and fitted curve between 1/r0 and 1/C0 at different initial concentrations (b) (Catalyst amount=1.0 g/L, pH=6.0)
Fig.6 Influence of initial pH on decolorization efficiency of MO (Catalyst amount=1.0 g/L, C0=10 mg/L)
3.3.4 Reuse of catalyst
The reuse of catalyst is one of the key steps to develop heterogeneous photocatalysis technology for practical implication. So it is essential to evaluate the stability and reuse of the catalyst associated with a significant cost savings of the treatment. As shown in Fig.7, the decolorization efficiencies for five cycles are 92.4%, 88.9%, 87.3%, 86.9% and 84.7%, respectively, after 60 min of visible light irradiation time. This shows a slight drop in efficiency, which is likely due to the fouling of the catalyst and the loss during filtration. It can be concluded that the reusability of AC/n-CdS/CS composite catalyst is revealed.
Fig.7 Reuse of catalyst on MO decolorization efficiency (Catalyst amount = 1.0 g/L, C0 =10 mg/L, pH = 6.0)
4 Conclusions
(1) Characterization results of AC/ nanosized CdS/CS (AC/n-CdS/CS) composites prepared by simulating bio-mineralization method indicate that hexagonal phase of CdS is formed on raw AC/CS and AC/n-CdS/CS composites exhibit better thermal stability than either raw CS or n-CdS/CS.
(2) The decolorization of MO by this innovative composite catalyst under visible light irradiation is synergistic effect between photocatalysis and adsorption. The decolorization of MO catalyzed by AC/n-CdS/CS fits with the Langmuir-Hinshelwood kinetics model and a surface reaction, where the dyes are absorbed, is the controlling step of the process.
(3) Decolorization efficiency of MO is improved with the increase in catalyst amount, while further increase in catalyst amount fails to result in obvious improvement in decolorization efficiency because of the screening effect. The photodecolorization of MO is more efficient in acidic media than alkaline media. The recycle and reuse experiments confirm the reusability of AC/n-CdS/CS composite catalyst.
References
[1] KIM Y M, LEE J Y, JEONG H R, LEE Y J, UM M H, JEONG K M, YEO M K, KANG M. Methyl orange removal over Zn-incorporated TiO2 photo-catalyst [J]. J Ind Eng Chem, 2008, 14(3): 396-400.
[2] AAL A A, BARAKAT M A, MOHAMED R M. Electrophoreted Zn-TiO2-ZnO nanocomposite coating films for photocatalytic degradation of 2-chlorophenol [J]. Appl Surf Sci, 2008, 254(15): 4577-4583.
[3] KAUR S, SINGH V. TiO2 mediated photocatalytic degradation studies of Reactive Red 198 by UV irradiation [J]. J Hazard Mater, 2007, 141(1): 230-236.
[4] BARAN W, MAKOWSKI A, WARDAS W. The effect of UV radiation absorption of cationic and anionic dye solutions on their photocatalytic degradation in the presence TiO2 [J]. Dyes Pigments, 2008, 76(1): 226-230.
[5] LI Jun-jie, LIU Shi-qing, HE Yu-yang, WANG Jia-qiang. Adsorption and degradation of the cationic dyes over Co doped amorphous mesoporous titania-silica catalyst under UV and visible light irradiation [J]. Microporous Mesoporous Mater, 2008, 115(3): 416-425.
[6] DUTTA P, FENDLER J H. Preparation of cadmium sulfide nanoparticles in self-replicating reversed micelles [J]. J Colloid Interface Sci, 2002, 247(1): 47-53.
[7] KUMAR A, MITAL S. Synthesis and photophysics of purine-capped Q-CdS nanocrystallites [J]. Photochem Photobiol Sci, 2002, 22(1): 737-741.
[8] NI T, NAGESHA D K, ROBLES J, MATERER N F, M?SSIG S, KOTOV N A. CdS nanoparticles modified to chalcogen sites: New supramolecular complexes, butterfly bridging, and related optical effects[J]. J Am Chem Soc, 2002, 124(15): 3980-3992.
[9] XU W, LIAO Y T, AKINS D L. Formation of CdS nanoparticles within modified MCM-41 and SBA-15 [J]. J Phys Chem B, 2002, 106(43): 11127-11131.
[10] WANG Cheng-yu, SHANG Hua-mei, TAO Ying, YUAN Tong-suo, ZHANG Guo-wu. Properties and morphology of CdS compounded TiO2 visible light photocatalytic nanofilms coated on glass surface [J]. Sep Purif Technol, 2003, 32(1/2/3): 357-362.
[11] TETSUYA K, GUAN G Q, AKIRA Y. LaMnO3/CdS nanocomposite: A new photocatalyst for hydrogen production from water under visible light irradiation [J]. Chem Phys Lett, 2003, 371(5/6): 563-567.
[12] WU L, YU J C, FU X Z. Characterization and photocatalytic mechanism of nanosized CdS coupled TiO2 nanocrystals under visible light irradiation [J]. J Mol Catal A: Chem, 2006, 244(1/2): 25-32.
[13] YI H, WU L Q, BENTLEY W E, GHODSSI R, RUBLOFF G W, CULVER J N, PAYNE G F. Biofabrication with chitosan [J]. J Biomacromol, 2005, 6(6): 2881-2894.
[14] RANGEL M J R, MONROY Z R, LEYVA R E, DIAZ F P E, SHIRAI K. Chitosan selectivity for removing cadmium(II), copper(II), and lead(II) from aqueous phase: pH and organic matter effect [J]. J Hazard Mater, 2009, 162(1): 503-511.
[15] AYDN Y A, AKSOY N D. Adsorption of chromium on chitosan: Optimization, kinetics and thermodynamics [J]. Chem Eng J, 2009, 151(1/2/3): 188-194.
[16] LI Zhan, DU Yu-min, ZHANG Zhi-ling, PANG Dai-wen. Preparation and characterization of CdS quantum dots chitosan biocomposite [J]. React Funct Polym, 2003, 55: 35-43.
[17] LI Zhan, DU Yu-ming. Biomimic synthesis of CdS nanoparticles with enhanced luminescence [J]. Mater Lett, 2003, 57(16): 2480-2484.
[18] FENG Duan, WANG Fang, CHEN Zi-lin. Electrochemical glucose sensor based on one-step construction of gold nanoparticle-chitosan composite film [J]. Sens Actuators B: Chem, 2009, 138(2): 539-544.
[19] ?LHAN U. Kinetics of the adsorption of reactive dyes by chitosan [J]. Dyes Pigments, 2006, 70(2): 76-83.
[20] KYZAS G Z, LAZARIDIS N K. Reactive and basic dyes removal by sorption onto chitosan derivatives [J]. J Colloid Interface Sci, 2009, 331(1): 32-39.
[21] ZHU Hua-yue, JIANG Ru, XIAO Ling, CHANG Yu-hua, GUAN Yu-jiang, LI Xiao-dong, ZENG Guang-ming. Photocatalytic decolorization and degradation of Congo Red on innovative crosslinked chitosan/nano-CdS composite catalyst under visible light irradiation [J]. J Hazard Mater, 2009, 169(1/2/3): 933-940.
[22] JIANG Ru, ZHU Hua-yue, LI Xiao-dong, XIAO Ling. Visible light photocatalytic decolourization of C. I. Acid Red 66 by chitosan capped CdS composite nanoparticles [J]. Chem Eng J, 2009, 152(2/3): 537-542.
[23] MATOS J, LAINE J, HERRMANN M. Effect of the type of activated carbons on the photocatalytic degradation of aqueous organic pollutants by UV-irradiated titania [J]. J Catal, 2001, 200(1): 10-18.
[24] ARANA J, DONA R J M, RENDON E T, CABO C G, GONZALEZ D O, HERRERA M J A, PEREZ P J, COLON G, NAVIO J A. TiO2 activation by using activated carbon as a support: Part I. Surface characterisation and decantability study [J]. Appl Catal B: Environ, 2003, 44(2): 161-172.
[25] LI You-ji, LI Xiao-dong, LI Jun-wen, YIN Jing. Photocatalytic degradation of methyl orange by TiO2-coated activated carbon and kinetic study [J]. Water Res, 2006, 40(6): 1119-1126.
[26] SOBANA N, SWAMINATHAN M. Combination effect of ZnO and activated carbon for solar assisted photocatalytic degradation of Direct Blue 53 [J]. Sol Energy Mater Sol Cells, 2007, 91(8): 727-734.
[27] LAINE M J, HERRMANN J, UZCATEGUI J M, BRITO D. Influence of activated carbon unpon titania on aqueous photocatalytic concecutive runs of phenol photodegradation [J]. Appl Catal B: Environ, 2007, 70(1/2/3/4): 461-469.
[28] XU D P, HUANG Z H, KANG F Y, INAGAKI M, KO T H. Effect of heat treatment on adsorption performance and photocatalytic activity of TiO2-mounted activated carbon cloths [J]. Catal Today, 2008, 139(1/2): 64-68.
[29] XU Jing-jing, AO Yan-hui, FU De-gang, YUAN Chun-wei. Synthesis of fluorine-doped titania-coated activated carbon under low temperature with high photocatalytic activity under visible light [J]. J Phys Chem Solids, 2008, 69: 2366-2370.
[30] SUN Jian-hui, WANG Xiao-lei, SUN Jing-yu, SUN Rui-xia, SUN Sheng-peng, QIAO Li-ping. Photocatalytic degradation and kinetics of Orange G using nano-sized Sn(IV)/TiO2/AC photocatalyst [J]. J Mol Catal A: Chem, 2006, 260(1/2): 241-246.
[31] DVORANOVAA D, BREZOVA V, MAZURA M, MALATIB M A. Investigations of metal-doped titanium dioxide photocatalysts [J]. Appl Catal B: Environ, 2002, 37(2): 91-105.
[32] SATHISH M, VISWANATH R P. Photocatalytic generation of hydrogen over mesoporous CdS nanoparticle: Effect of particle size, noble metal and support [J]. Catal Today, 2007, 129(3/4): 421-427.
[33] SUN L P, DU Y M, YANG J H, SHI X W, LI J, WANG X H, KENNEDY J F. Conversion of crystal structure of the chitin to facilitate preparation of a 6-carboxychitin with moisture absorption-retention abilities [J]. Carbohydr Polym, 2006, 66(2): 168-175.
[34] SREENIVASAN K. Thermal stability studies of some chitosan metal ion complexes using differential scanning calorimetry [J]. Polym Degrad Stab, 1996, 52(1): 85-87.
[35] NEPPOLIAN B, CHOI H C, SAKTHIVEL S, ARABINDOO B, MURUGESAN V. Solar light induced and TiO2 assisted degradation of textile dye reactive blue 4 [J]. Chemosphere, 2002, 46(2): 1171-1181.
[36] YOSHIDA H, OKAMOTO A, KATAOKA T. Adsorption of acid dye on crosslinked chitosan fibers: Equilibria [J]. Chem Eng Sci, 1993, 48(12): 2267-2272.
Foundation item: Project(21007044) supported by the National Natural Science Foundation of China; Project(20050532009) supported by the Doctoral Foundation of Ministry of Education of China
Received date: 2009-12-12; Accepted date: 2010-02-04
Corresponding author: JIANG Ru, PhD; Tel: +86-15867636396; E-mail: jiangru0576@163.com
- Synergy of adsorption and visible light photocatalysis todecolor methyl orange by activated carbon/nanosized CdS/chitosan composite
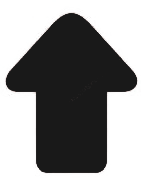