- Abstract:
- 1 Introduction▲
- 2 Model design and test o...▲
- 3 Damage characteristics▲
- 4 Result and analysis▲
- 5 Conclusions▲
- References
- Figure
- Fig.1 Geometry and reinforcement details of frame (unit: mm)
- Fig.2 Test setup for application of lateral force
- Fig.3 Final damage pictures of the first floor column in axes A (a) and B (b)
- Fig.4 Hysteresis curves of frame top layer
- Fig.5 Restoring force model of frame
- Fig.6 Curves of stiffness degeneration of every storey of frame: 1—The first storey stiffness under positive loading; 2—The second storey stiffness under positive loading; 3—The third storey stiffness under positive loading
- Fig.7 Hysteresis loop of frame under cycle i
J. Cent. South Univ. Technol. (2010) 17: 1364-1369
DOI: 10.1007/s11771-010-0643-9
Frame property of unequal storey height with specially shaped columns under cyclic loading
WANG Tie-cheng(王铁成)1, ZHANG Xue-hui(张学辉)2
1. School of Civil Engineering, Tianjin University, Tianjin 300072, China;
2. School of Civil Engineering, Hebei University of Science and Technology, Shijiazhuang 050018, China
? Central South University Press and Springer-Verlag Berlin Heidelberg 2010
Abstract:
A 1/3-scale reinfored concrete (RC) frame of unequal storey height with specially shaped columns was tested under low frequency cyclic loading. The damage characteristic, bearing capacity, deformation capacity and ductility were analyzed. The restoring force model of the frame was obtained based on the study of the hysteresis curve measured in experiment, and the stiffness degeneration characteristics of every storey of the frame were analyzed. Finally the accumulated damage was analyzed with the damage assessment model. It is shown that the seismic behavior of this frame of unequal storey height with specially shaped columns is generally good, but the bottom of first floor column is a weak part, which should be paid more attention in design, and the restoring force model derived from this experiment can be seen as a valuable guide for design and non-linear finite element analysis for this kind of structure.
Key words:
frame; specially shaped column; restoring force; accumulated damage; seismic behavior;
1 Introduction
The specially shaped columns are the columns with sections of ‘L’ shape, ‘T’ shape or cross shape, in which the ratio of section height to section thickness of column limb is less than 4. The concrete frame or frame-shear wall structure using specially shaped columns is called structure with specially shaped columns [1]. Using structure with specially shaped columns could make the furniture layout more easily, and edges and corners disappear in the room unlike that with rectangular column. So, the structure with specially shaped columns has good prospect use in building. Scholars have done great amount experimental research and theory analysis to reinforced concrete (RC) frame and components with specially shaped columns [2-9]. But the research on RC frame with specially shaped columns in high seismic intensity zone is not enough, and in actual project there are some structures whose the bottom storey height is higher than other stories height because of functional requirement, and the research on experiment and theory for this RC frame with specially shaped columns is less. To promote the application for the frame of unequal storey height with specially shaped columns in high seismic intensity, a 1/3-scale frame of unequal storey height with specially shaped columns was designed according to the specification for specially shaped columns under low frequency cyclic loading to simulate the stress condition of district eight and site Ⅵ, the damage characteristic, bearing capacity, deformation capacity and ductility were analyzed, the restoring force model of the frame was obtained based on the study of the hysteresis curve, and the stiffness degeneration characteristics of every storey of the frame were analyzed, also the cumulated damage was analyzed with the damage estimating model.
2 Model design and test overview
The model is a 1/3-scale frame with specially shaped columns, which is two spans-three stories with unequal storey height. Geometry and reinforcement details of frame model are shown in Fig.1. The grades of longitudinal bars in the first two storey framework beams and each column are HRB400, the grades in the third beams are HRB335, and the grades of stirrups in all beams and columns are HPB235. The strength grade of concrete is C45, cube strength after 28 d is 52.6 MPa, and elastic modulus of concrete is 36.7 GPa.
Test loading device of frame model is shown in Fig.2. The vertical load is imposed by there vertical hydraulic jacks: the force imposed in middle column is 100 kN, and those in two edge columns are 72 kN. A tension-pressure horizontal jack is set at the beam end of top storey, and a level displacement gauge is put on each beam end on another side of frame, the loading- displacement data are gathered by acquisition system DH3818. Cracks are observed by artificial.
Fig.1 Geometry and reinforcement details of frame (unit: mm)
Fig.2 Test setup for application of lateral force
The loading system is controlled jointly by force and displacement, so, control condition of loading is based on the force before the yield of frame, in every loading level the force is cycled once. Yield displacement of the frame Δy in experiment is the corresponding displacement as any one steel yielding in beam, after structure yielding the control condition of loading is based on yield displacement of integer times. In each loading level the force is cycled there times. The structure is thought to be damaged when the level thrust drops to 85% of maximum load.
3 Damage characteristics
Cracking and damage characteristics of concrete in structure were observed. The cracks in beams were wide and extended to oblique top in beams, and cracks in beams were wider than those in columns. The concrete protective coating at beam end damaged seriously, there were peels in some beam end at late loading. The vertical steel in beam was exposed. There was a very obvious vertical crack in the junction of beam and the core of joint. There were some shear oblique cracks that appeared in some core of joint at middle stage loading, but the appearance order was later than that of the crack in beam and column, and the oblique cracks did not continue at late loading. At late loading the concrete of the bottom of first floor column in every axis was crushed and peeled seriously, vertical steel at the bottom of the first floor column was bended to lantern shape, and concrete crack at the bottom of other floor columns was less than that of the first floor. It can be seen from the final damage that stiffness of the first floor was weak obviously because the first floor height was higher than other floor height of this structure with specially shaped columns, which made the concrete in web at the bottom of first floor column crushed and peeled easily, and vertical steel in the bottom of first floor column was bended possibly, the degree of damage at the first floor column was much more serious compared with that of beam. So, it is necessary to take measures to enhance the side lateral stiffness of the first floor for this frame of unequal storey height with specially shaped columns. The final damage pictures of first floor column in axes A and B are given in Fig.3.
4 Result and analysis
4.1 Hysteresis characteristics
The hysteresis curves of horizontal force (F) and horizontal displacement (U3) for the third floor are shown in Fig.4.
The shape of hysteresis curve was spindle shape at beginning. After yielding of tension steel in the frame with specially shaped columns, with the increase of cyclic loading time the total displacement increased continuously, but the change of force was small, and the shape of hysteresis curve became bow shape gradually. In every loading process, the slope of loading curve became lower with the increase of loading. An anti-point bending appeared in the loading curve after several times cyclic loading and it had tendency of pinch together in the middle part, which reflected that the influence of steel slip anchor was gradually aggravated with the increase of displacement. The unloading curve was relatively steep at beginning unloading, and the curve was slowly flat with the decrease of loading. The speed with resumption of deformation gradually quickened, which proved the resumption of deformation lag. The slope of hysteresis curve became reducing slowly with the growth of times cyclic loading and unloading, and the unloading stiffness of structure became degradation. There was residual deformation in structure after the load of zero, and the residual deformation increased constantly with the growth of times of cyclic loading and unloading. From the measured hysteresis curve it can be seen that the hysteresis loop about this structure of unequal storey height with specially shaped columns was relatively full, and through relatively the equivalent viscous damping coefficient also proved that the frame had higher dissipation energy capacity.
Fig.3 Final damage pictures of the first floor column in axes A (a) and B (b)
Fig.4 Hysteresis curves of frame top layer
4.2 Restoring force model
The restoring force model of frame is the capability for restoring original deformation after unloading. Restoring force characteristics curve reflects the features about strength, stiffness, deformability, stiffness degradation, and energy dissipation of the structure or components under cyclic loading. Because the hysteresis curve measured in experiment is rather complex, it is simulated by sub-linear straight line [10-11].
The restoring force model of frame was given by simplifying the hysteresis curve. In this work restoring force model was gotten to adopt multi-line model. The specific method was as follows: the points of yield load and flexible load are coincided; restoring force curve is a three-broken line considering yield load and maximum load; and stiffness degradation after maximum value, the unloading stiffness of every control points before maximum load are basically identical, and change with the variation of displacement after maximum load. According to the above assumptions the restoring force model is given in Fig.5. This restoring force model can be used as a base for non-linear finite element analysis for the frame structure of unequal storey height with specially shaped columns.
4.3 Stiffness degeneration
Stiffness is also an important indicator for seismic behavior of structure like bearing capacity and ductility. The total level earthquake magnitude is in direct proportion to the square root of anti-push stiffness of structures, that is to say, if the anti-push stiffness of building is big, then the natural cycle of the building is small, and the total level earthquake magnitude increases. On the other hand the total level earthquake magnitude reduces. But if the anti-push stiffness of building is too small, although the earthquake magnitude reduces, the sway of whole structure will increase too much, which is adverse for the structure. The stiffness of structure will gradually reduce under earthquake. When the stiffness is degenerated, the internal force of structure will be redistributed, the bearing capacity will be changed, and also deformation will be changed, so the stiffness degeneration of structure should be considered in design. The equivalent stiffness (K) is defined to the slope of connecting line from origin of coordinates to peak loading in a cycle that is the point in skeleton curve. It is computed from K=F/U, where F is the loading and U is the displacement. With increasing the displacement, the stiffness of structure in a cycle is smaller than that in front cycle, so the stiffness will be reduced with the increase of cycle and deformation, which is stiffness degeneration. In general, it is expressed by equivalent stiffness. The curves of stiffness degeneration of the frame are given in Fig.6.
Fig.5 Restoring force model of frame
Fig.6 Curves of stiffness degeneration of every storey of frame: 1—The first storey stiffness under positive loading; 2—The second storey stiffness under positive loading; 3—The third storey stiffness under positive loading
It can be seen from the curves of stiffness degeneration in each storey that initial stiffness of the first storey under positive and negative loading is bigger than that of the second storey and the third storey. Under positive loading the initial stiffness of the first storey is higher 3.874 kN/mm than that of the second storey, and 4.957 kN/mm than that of the third storey; under negative loading it is higher 5.037 kN/mm than that of the second storey, and 5.381 kN/mm higher than that of the third storey; initial stiffness of the second storey under positive and negative loading are also bigger than that of the third storey. With increasing the deformation, the stiffness of every storey gradually degenerates, stiffness degeneration rate of the first storey is faster than that of the second storey and third storey at the beginning and middle loading, at least the stiffness of every storey becomes flat gradually. The stiffness degeneration course of every storey experiences stiffness falling quickly, and stiffness descends medially and slowly there stage.
4.4 Accumulated damage
The concrete structure will have serious injuries or even collapse because of great plastic deformation under strong earthquake, so, the safe and reasonable anti- seismic structure should be designed according to damage forecast value of earthquake fortified intensity [12-13]. Based on the principle of energy dissipation and the loading-displacement hysteresis curve of RC structure under low frequency cyclic loading, the power worked by external force under no injuries ideal state of structure as to initial scalar, to build damage assessment model for the structure under cyclic loading, this model could comprehensively response the accumulated damage characteristics such as energy dissipation, intensity degradation and stiffness degradation of concrete structure in process of deformation under cyclic loading. It could track the degree of deterioration accumulated damage expressed by accumulated damage index Di of the structure under any cycle to response the actual damage of the concrete structure [14]. This test model is a relatively ruled frame structure, so according to Ref.[14] the accumulated damage index can be computed using the following formula:
(1)
The meaning of parameters in formula (1) can be explained from Fig.7, in which K0 is the initial stiffness of structure, f1(Δni) and f2(-Δni) are the shear functions of the basement under positive and negative loading at cycle i, and ±Δni are the top Layer absolute displacements at positive and negative peak point at cycle i. According to the power worked by external force and conservation of energy law, the accumulated damage index can also be computed as:
(2)
where S is the area of the graphics.
Fig.7 Hysteresis loop of frame under cycle i
The computed results of accumulated damage index in main stage are listed in Table 1. Researchers proved that the structure with specially shaped columns could be divided to five injury stages according to accumulated damage index and the actual damage extent of the structure: (1) accumulated damage index Di=0-0.2, the structure is basically intact; (2) Di=0.2-0.4, the structure is minor injured, and the crack width is in the range of 0.2 mm; (3) Di=0.4-0.6, the structure is medium injured, and the crack width is in the range of 0.2 mm to yield. (4) Di=0.6-0.9, the load is from yield to damage, structure is seriously damaged; and (5) Di>0.9, the structure collapses [14]. It can be seen from Table 1 that the accumulated damage index in main loading stage of this frame with specially shaped columns could conform to the five injury stages in Ref.[14].
The values of loading, displacement in main stage and displacement ductility coefficient μ of the frame are also listed in Table 1. The displacement ductility coefficient was obtained by damage displacement dividing yield displacement, and the yield displacement was obtained by adopting equal area law on skeleton curve. The damage displacement was the displacement when unloading value drops to 85% of limit loading corresponding displacement. Load-bearing capacity and ductility are the important indicators in seismic engineering. Research shows that the value of structural displacement ductility coefficient is in the range of 3-5 to guarantee that the structural components have enough curvature ductility. Thus, it is more conducive for the anti-seismic structure [15]. The average of displacement ductility coefficient computed for this frame with specially shaped columns is 3.60, which is in the range of 3-5, indicating that the ductility of this structure is good, and the structure has high anti-seismic capacity.
Table 1 Loading, displacement and accumulated damage index of frame in main stage
5 Conclusions
(1) The bottom of the first floor column is a weak part for this frame of unequal storey with specially shaped columns, concrete and vertical steel in web at the bottom of the first floor column are damaged more seriously than that at beam end. It is shown that the frame of unequal storey height with specially shaped columns building in high-intensity earthquake zone should be paid more attention to enhance lateral stiffness of the first floor column to avoid serious damage.
(2) The average of displacement ductility coefficient is 3.60. Hysteresis curve is fuller and the frame has enough energy capacity, so this structure of unequal storey height with specially shaped columns has good ductility and anti-seismic capability.
(3) By comparing the curves of stiffness degeneration of every storey, the initial stiffness of the first storey in loading is bigger than that of the second and third stories obviously. Columns in the first storey could withstand more earthquake magnitude than those of second and third storey, but with the increase of the deformation, the stiffness degeneration rate of the first storey is faster than that of the second and third stories, at last the stiffness of every storey is equal gradually. So, enhancing the stiffness of the first storey could avoid the stiffness degeneration rate of the first storey too fast, which could improve the anti-seismic capability of the frame with specially shaped columns.
(4) Restoring force model of frame obtained from test could give a reference to engineering design and nonlinear finite element analysis for the structure of unequal storey height with specially shaped columns.
References
[1] JGJ 149—2006. Technical specification for concrete structure with specially shaped columns [S]. Beijing: China Architectural Industry Press, 2006.
[2] SZALAI J, PAPP F. A new residual stress distribution for hot-rolled I-shaped sections [J]. Journal of Constructional Steel Research, 2005, 61(6): 845-861.
[3] CHEN C C, LI J M, WENG C C. Experimental behaviour and strength of concrete-encased composite beam–columns with T-shaped steel section under cyclic loading [J]. Journal of Constructional Steel Research, 2005, 61(7): 863-881.
[4] WANG Tie-cheng, LI Xin-hua, ZHAO Shao-wei. Seismic behavior of frame with specially shaped columns subjected to cyclic load [J]. Transactions of Tianjin University, 2007, 13(5): 340-343.
[5] KIM S D, KIM S S, JU Y K. Strength evaluation of beam–column connection in the weak axis of H-shaped column [J]. Engineering Structures, 2008, 30(6): 1699-1710.
[6] XU Yu-ye, WU Bo. Fire resistance of reinforced concrete columns with L-, T-, and +-shaped cross-sections [J]. Fire Safety Journal, 2009, 44(6): 869-880.
[7] WU Bo, XU Yu-ye. Behavior of axially-and-rotationally restrained concrete columns with ‘+’-shaped cross section and subjected to fire [J]. Fire Safety Journal, 2009, 44(2): 212-218.
[8] WANG Tie-cheng, ZHANG Xue-hui, KANG Gu-yi. Experimental comparison of seismic behavior of two RC frames with specially shaped columns [J]. Journal of Tianjin University, 2007, 40(7): 791-798. (in Chinese)
[9] WANG Tie-cheng, ZHANG Xue-hui, ZHAO Hai-long. Experimental study on seismic behavior of RC frames with specially shaped columns reinforced by fiber [J]. Journal of Earthquake Engineering and Engineering Vibration, 2008, 28(6): 178-185.
[10] L? Xi-lin, LU Wei-dong. Experimental research of square-steel tube concrete columns subjected to cyclic loading [J]. Journal of Building Structure, 2000, 21(2): 2-7.
[11] XU Ya-feng, TANG Hong, CHEN Zhao-cai, WANG Lian-guang. Research of resilience model of steel high strength concrete frame node [J]. Journal of Lanzhou Technology University, 2004, 30(5): 116-118. (in Chinese)
[12] FALEIRO J, OLLER S, BARBAT A H. Plastic–damage seismic model for reinforced concrete frames [J]. Computers and Structures, 2008, 86: 581-597.
[13] FANG S E, RICARDO P, de ROECK G. Damage identification of a reinforced concrete frame by finite element model updating using damage parameterization [J]. Journal of Sound and Vibration, 2008, 313: 544-559.
[14] DIAO Bo, LI Shu-chun, YE Ying-hua. Analysis and experiment of cumulated damage of RC structures with special columns under cyclic loading [J]. Journal of Building Structures, 2008, 29(1): 57-63. (in Chinese)
[15] ZHAO Yan-jing, LI Zhong-xian, CHEN Yun-xia. Research on limit values of axial compression ratios of specially shaped RC columns in case of 4th seismic grade [J]. Journal of Building Structures, 2004, 25(3): 58-62. (in Chinese)
Foundation item: Project(50878141) supported by the National Natural Science Foundation of China; Project(Z2010250) supported by the Natural Science Foundation of Education Department of Hebei Province, China
Received date: 2010-01-05; Accepted date: 2010-04-08
Corresponding author: ZHANG Xue-hui, PhD; Tel: +86-311-81668900; E-mail: zhxh1999@126.com
- Frame property of unequal storey height withspecially shaped columns under cyclic loading
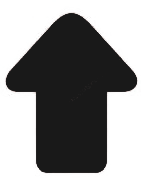