- Abstract:
- 1 Introduction▲
- 2 Experimental▲
- 3 Results and discussion▲
- References
- Figure
- Fig.1 UV-Vis spectra of complex catalyzed by FeTSPc with pH ranging from 0.5 to 4.0
- Fig.2 Effect of concentration of aniline on rate of aniline polymerization catalyzed by FeTSPc
- Fig.3 Effect of concentration of SDS on rate of aniline polymerization catalyzed by FeTSPc
- Fig.4 Effect of concentration of H2O2 on rate of aniline polymerization catalyzed by FeTSPc
- Fig.5 Effect of reaction time on polymerization rate of aniline catalyzed by FeTSPc
- Fig.6 UV-Vis spectra change of PANI/SDS complex during titration by 1.0 mol/L NaOH and 1.0 mol/L HCl in pH range from 1.0 to 11.0 (a) and from 11.0 to 1.0 (b)
- Fig.7 CV of PANI/SDS complexes recorded in 1.0 mol/L HCl with scan rate of 100 mV/min
- Fig.8 FT-IR spectra of PANI/SDS complex and PANI
- Fig.9 TG, DTA and DTG curves of PANI/SDS complex
J. Cent. South Univ. Technol. (2009) 16: 0743-0748
DOI: 10.1007/s11771-009-0123-2
Iron(Ⅱ) tetrasulfophthalocyanine mimetic enzymatic synthesis of
conducting polyaniline in micellar system
HU Xing(胡 兴)1,2, LIU Hui(刘 惠)1,2, ZOU Guo-lin(邹国林)3
(1. Department of Life Sciences, Huaihua University, Huaihua 418008, China;
2. Key Laboratory of Research and Utilization of Ethnomodicinal Plant Resources of Hunan Province,
Huaihua 418008, China;
3. College of Life Sciences, Wuhan University, Wuhan 430072, China)
Abstract:
Iron(Ⅱ) tetrasulfophthalocyanine (FeTSPc), as a novel mimetic enzyme of peroxidase, was used in the synthesis of a conducting polyaniline (PANI)/sodium dodecylsulfate (SDS) complex in SDS aqueous micellar solutions. The effects of pH, concentrations of aniline, SDS and H2O2, and reaction time on polymerization of aniline were studied in this case as shown by UV–Vis absorption spectroscopy. The results show that a wide range of pH (0.5-4.0) is required to produce the conducting PANI, and the optimal pH is 1.0 in SDS micelle. The optimal concentrations of aniline, SDS and H2O2 in feed, and reaction time in this case for the production of conducting PANI are respectively 10 mmol/L, 10 mmol/L, 25 mmol/L, and 15 h. FT-IR spectrum, elemental analysis, conductivity, cyclic voltammetry and thermogravimetric analysis confirm the thermal stability and electroactive form of PANI.
Key words:
iron(Ⅱ) tetrasulfophthalocyanine; polyaniline; mimetic enzyme; conductivity; micelle;
1 Introduction
Recently, enzymes like catalysts have been widely used in the synthesis of conducting polyaniline (PANI) for their environment benign reaction conditions, a higher degree of kinetic control over the reaction and a higher yield of product. For example, AKKARA et al [1] investigated the horseradish peroxidase (HRP) used for the oxidative polymerization of aniline to synthesize a conducting PANI in the presence of hydrogen peroxide at about pH 4.3. SAKHAROV et al [2-4] reported that the optimal pH of laccase and palm tree peroxidase which were used for the synthesis of polyelectrolyte complex of PANI and sulfonated polystyrene was 3.5. HU et al [5-7] also studied the hemoglobin (Hb) as mimetic peroxidase used for the synthesis of conducting PANI in the sodium dodecylsufate (SDS) micellar solutions and polyelectrolyte solutions at pH of 2.0.
Although HRP, laccase, palm tree peroxidase and Hb are promising biocatalytic approaches to the synthesis of PANI,unfortunately, they all showed low activity towards aniline and low stability at low pH in the synthesis of conducting PANI, and natural enzymes are expensive. Therefore, it is necessary to develop a cheap, high efficient and stable mimetic enzyme catalyst to replace the native enzyme and protein.
Some metalloporphyrins and metallophthalocyanine (MPc) show similar catalytic function as peroxidase [8]. These two complexes have a similar structure. Metalloporphyrins were insoluble in the acidic solutions. Thus porphyrins were not effective mimetic enzymes to polymerize aniline in low pH aqueous conditions. Presently, MPc complexes as catalysts have been paid considerable attention [9-13], because they are more stable in degradation, cheaper and more readily available than porphyrins. And Iron(Ⅱ) tetrasulfophthalocyanine (FeTSPc) was found to be an efficient catalyst with higher turnover rates, more soluble and cheaper [13].
Furthermore, it was found that SDS micelles could provide suitable local environment, leading to the formation of the conducting PANI. These systems can form charge double layers around which counter ions such as H+, may be condensed and the monomers may associate [14]. Negative surfactants such as SDS manipulated the solubility and process ability of enzymatically synthesized conducting PANI as good dopants [15].
In this work, FeTSPc, because of its higher solubility, stability, efficiency and lower price, was used to synthesize a conducting PANI/SDS complex in the SDS micellar system, and the details of the optimal conditions and characterization of PANI were discussed in this system.
2 Experimental
2.1 Materials
FeTSPc was synthesized according to Ref.[16]. Sodium dodecylsufate (ultra pure, SDS) was purchased from Sigma Chemicals Co., USA. Aniline monomer (purity 99.5%) and hydrogen peroxide (above 30%, mass fraction) were purchased from Shanghai Chemical Agent, China. Aniline was purified by distillation before use. Other chemicals and solvents were of analytical grade or better and used as received.
2.2 FeTSPc mimetic enzymatic polymerization of aniline
2.2.1 Experimentations of FeTSPc mimetic enzymatic kinetics
To test the effects of pH, aniline, SDS and H2O2, the polymerization of aniline was carried out by FeTSPc- catalyzed in 0.2 mol/L Na2HPO4-citric acid buffer solutions (pH 0.5-4.0) at 25 ℃. The concentration of aniline, SDS and H2O2 in feed varied from 0.55 to 100 mmol/L, from 2 to 100 mmol/L and from 5 to 75 mmol/L, respectively. The mimetic enzymatic reaction was initiated by adding FeTSPc (100 mmol/L). The polymerization of aniline was evaluated using UV-Vis spectrophotometer. The spectra were recorded 15 h after the polymerization initiates.
2.2.2 Synthesis of PANI/SDS complexes
The reaction was carried out by FeTSPc-catalyzed in a 100 mL, 0.2 mol/L Na2HPO4-citric acid buffer solutions maintained at pH 1.0, which contained aniline (10 mmol/L) and SDS (10 mmol/L) at 25 ℃. 58 mg SDS was added first to the buffer solution, followed by an addition of aniline under constant stirring, then 0.1 mL of 10 g/L FeTSPc stock solution (100 mmol/L) was added. The reaction was initiated by the addition of a stoichiometric amount of H2O2 (25 mmol/L) under vigorous stirring. After adding H2O2, the reaction was left stirring for 15 h to complete the polymerization, and acetone was added to collapse the micelles and precipitate the synthesized PANI/SDS complex from the solution. The synthesized PANI/SDS complex was collected by centrifugation and washed with 50% acetone/water mixture to remove the aniline monomer and the oligomer. The final purified polymer was dried under vacuum at 50℃ for 24 h for further characterization.
2.3 Spectroscopic methods
The UV-Vis spectra were obtained using a Cary 100 UV-Vis spectrophotometer (Varian, USA). In every measurement, distilled water was used as a control. The FT-IR measurements were carried out using a Nicolet 170SX FT-IR spectrophotometer in the form of KBr pellets.
2.4 Cyclic voltammograms
Cyclic voltammograms (CVs) were carried out with a computer-controlled electrochemistry workstation (CHI, USA). An electrochemical cell with the standard two compartments and a conventional three-electrode configuration were used for electrochemical experiments. CV was recorded by using a three-electrode cell with the thin film cast from DMF solution of the PANI/SDS complex on a platinum plate (CHI, d2 mm) as the working electrode, a saturated calomel electrode(SCE) as the reference electrode was immersed in the electrolyte via a Luggin capillary situated at the tip of the working electrode and a platinum gauze as a counter electrode. The CV was obtained at room temperature in a 1.0 mol/L HCl electrolyte solution by scanning between -0.2 and 1.0 V at a scan rate of 100 mV/min.
2.5 Others characterization methods
Elemental analysis was carried out using a VarioEL Ⅲelementar analysensystem (Germany). Thermo- gravimetric analysis (TGA) was performed using a Netzsch STA 449C thermogravimetric analyzer (Germany). The conductivity of the PANI/SDS complex was measured using a DDSJ-308 electrometer (Shanghai Jing Ke Rex, China).
3 Results and discussion
3.1 FeTSPc mimetic enzymatic polymerization
3.1.1 Effect of pH on polymerization
The absorption spectra of PANI with SDS using FeTSPc mimetic enzymatic synthesis at pH 0.5 to 4.0 are given in Fig.1. In this pH range, the polymer shows that typical strong absorption bands at approximately 400 and 800 nm are due to the well-known polaron transition
Fig.1 UV-Vis spectra of complex catalyzed by FeTSPc with pH ranging from 0.5 to 4.0
[17]. The intensity of the absorption bands at 760 nm in pH range from 0.5 to 4.0 suggests that the extent of the polymerization is the highest at pH 1.0 and lowest at pH 4.0. These results show that the optimum pH of the polymerization reaction catalyzed by FeTSPc in SDS is 1.0, which is quite different from the PANI formed by peroxidase-catalyzed polymerization carried out at pH 4.3 (HRP) [14, 18-21], pH 3.5-4.4 (laccase and palm tree peroxidase) [2-4] and pH 2.0 (Hb) [5-7]. However, it is not a favorable condition because peroxidase and Hb are inactivated rapidly at pH 1.0. Therefore, FeTSPc is an efficient and advantageous catalyst for the synthesis of PANI/SDS complex in comparison with peroxidase and Hb.
A control experiment was also carried out to confirm that FeTSPc catalysis was responsible for the polymerization. When only FeTSPc (without H2O2) or without both (FeTSPc and H2O2) is added to the solution, no polymerization of aniline will occur. And when only H2O2 (without FeTSPc) is added to the solution, the polymerization of aniline will occur feebly. In contrast, if both FeTSPc and H2O2 are added, obviously, the polymerization of aniline will occur. This control experiment is strong evidence that the polymerization of aniline owes to FeTSPc catalysis in the presence of H2O2.
3.1.2 Effect of concentration of aniline and SDS on polymerization
In order to value the effect of aniline concentration on the polymerization rate, the kinetics of the reaction in which the aniline concentration varied from 0.55 to 100 mmol/L was studied. As can be seen from Fig.2, the increase of aniline concentration up to 10 mmol/L in feed results in the growth of the polymerization rate. However, a further increase of aniline concentrations causes the substrate to inhibit the catalysis of FeTSPc. Therefore, the polymerization of aniline catalyzed by FeTSPc is similar to that by peroxidase in this case, and the optimal
Fig.2 Effect of concentration of aniline on rate of aniline polymerization catalyzed by FeTSPc
concentration of substrate is 10 mmol/L.
In order to evaluate the effect of aniline and SDS concentrations on the polymerization, the kinetics of the reaction varied with the aniline and SDS concentrations from 2 to 100 mmol/L was investigated. In Fig.3, with the substrate concentration increasing to 10 mmol/L, the rate of transformation of substrate also increases. However, a further increase of the substrate concentrations leads to the decrease of the rate of transformation of the substrate. It is possible that under this reaction condition, with increasing SDS concentration (2-10 mmol/L), the micelles form in the solution when the concentration is over the critical micelle concentration (CMC) (8.3 mmol/L), and the polymerization rate increases. However, a further increase of aniline and SDS concentrations causes the substrate to inhibit FeTSPc, and the polymerization rate drops. Therefore, the maximal polymerization rate of aniline is observed at the concentrations of aniline and SDS of 10 and 10 mmol/L, respectively. And this concentration is chosen in all the further experiments.
Fig.3 Effect of concentration of SDS on rate of aniline polymerization catalyzed by FeTSPc
3.1.3 Effect of concentration of H2O2 on polymerization
H2O2 concentration also affects the polymerization rate. Comparison of absorbance at 760 nm in the presence of different concentrations of H2O2 shows that the yield of the aniline polymerization increases with the growth of H2O2 concentrations in feed up to 25 mmol/L, and the initial rate of mimetic enzymatic polymerization increases (Fig.4). However, at higher concentration of H2O2, the rate drops slowly. Evidently, the optimal concentration of H2O2 is 25 mmol/L because when the concentration of H2O2 is higher than its own optimal concentration, some side reactions proceed. The product of the side reaction is the active intermediate of FeTSPc catalytic cycle. However, its activity is lower than that of normal intermediate product by FeTSPc catalysts.
Fig.4 Effect of concentration of H2O2 on rate of aniline polymerization catalyzed by FeTSPc
Therefore, H2O2 is the suicide substrate for FeTSPc. The higher the concentration of H2O2 is, the faster the FeTSPc becomes blunt.
3.1.4 Effect of reaction time on polymerization
Fig.5 shows that reaction time affects the poly- merization rate by FeTSPc in SDS micelle. Comparison of values of absorbance at 760 nm for different time indicates that the polymerization rate increases with the extension of the reaction time. When the reaction time rises to 15 h, the absorbance at 760 nm reaches the maximum. However, a further extend of reaction time fails to achieve a conspicuous change of the values of absorbance at 760 nm. Thus, the optimal reaction time of the polymerization of aniline catalyzed by FeTSPc is 15 h.
Fig.5 Effect of reaction time on polymerization rate of aniline catalyzed by FeTSPc
3.2 Dedoping and redoping
To determine the reversible dedoping and redoping behaviors of the PANI/SDS complex, the absorption spectra of a complex prepared at pH 1.0 were studied with the change of pH. Fig.6(a) gives the change of absorption spectra of the complex with increasing pH from 1.0 to 11.0 by titrating with 1.0 mol/L NaOH. At pH 1.0, the PANI in the complex is in the doped state as reflected by the presence of the polaron band transition at about 400 and 800 nm [19, 22-23]. As the pH of the complex increases, the polaron bands in the region of 760-820 nm gradually disappear, but a strong absorption due to the exciton transition of the quinoid rings at 560-630 nm begins to emerge and increase, accompanied by a color change in the complex from green to blue (pH 9.2), and finally purple. Evidently, the PANI is fully dedoped to the emeraldine base form [19, 24]. The dedoped PANI can be redoped by titrating with 1.0 mol/L HCl. A reversible color change is observed, and the spectra are given in Fig.6(a). The dedoping and redoping reversibility induced by pH confirms the presence of the electroactive form of PANI in the PANI/SDS complex.
Fig.6 UV-Vis spectra change of PANI/SDS complex during titration by 1.0 mol/L NaOH and 1.0 mol/L HCl in pH range from 1.0 to 11.0 (a) and from 11.0 to 1.0 (b)
3.3 Cyclic voltammetry
The electrochemical property of the FeTSPc- catalyzed synthesized PANI/SDS complex is characterized by CV. Fig.7 shows the CV of the films of PANI/SDS complexes. Two well-resolved sets of peaks are observed with the complex corresponding to two reversible redox processes at 0.42 and 0.76 V (vs SCE) at a scan rate of 100 mV/min over a potential window of -0.2-1.0 V. By comparing these results of CV of PANI/SDS complex with those reported from literatures such as CV of PANI produced enzymatically by HRP [19], PEG-hematin [25], laccase [2] and palm tree peroxidase [4] or electrochemically [26], this study finds out that the results are identical. These recorded CV curves suggest that the FeTSPc catalytic synthesized PANI is electrochemically active.
Fig.7 CV of PANI/SDS complexes recorded in 1.0 mol/L HCl with scan rate of 100 mV/min
3.4 Elemental analysis
The results of elemental analysis of PANI/SDS complex synthesized at pH 1.0 show that contents of carbon, hydrogen, nitrogen, and sulfur are 43.87%, 6.80%, 3.39%, and 3.63%, respectively. It is evident that PANI is doped with SDS. The yield of PANI/SDS complex synthesized by FeTSPc at pH 1.0 is 86%. The conductivity of PANI/SDS complex, synthesized at pH 1.0 is determined to be of the order of 10-1 S/cm without addition of any external dopants.
3.5 FT-IR spectrum analysis
Fig.8 shows the FT-IR spectra of PANI/SDS complex and PANI in the region from 4 000 to 400 cm-1. Comparison of these spectra shows the existence of some bands in the spectrum of the complex characteristic for PANI. The bands at around 1 560 and 1 483 cm-1 are assigned to a C=C ring stretching in quinoid and benzenoid units of PANI in doped form [14-15] shifted to lower wave numbers from 1 584 and 1 493 cm-1, respectively. The presence of the characteristic peaks at 1 295 and 1 235 cm-1 for caromatic-N stretching vibration of linking with quinone and benzene ring secondary aromatic amine confirms that PANI in the complex is in doped form [15], because for undoped PANI the same peak is revealed at 1 310-1 313 cm-1 [18]. The C—H out-of-plane bending located at 795 cm-1 is due to a para-substitution pattern, indicating that a head-to-tail coupling of aniline occurs during the polymerization [19]. In addition, the peaks at
and
due to the asymmetric and symmetric S=O stretching and SO2 scissoring respectively, in the PANI/SDS spectra, confirmed the presence of the SDS in the complex [18].
Fig.8 FT-IR spectra of PANI/SDS complex and PANI
3.6 Thermal analysis
Thermogravimetric analysis (TGA) measurement of FeTSPc-catalyzed polymerization of PANI/SDS was performed under a N2 atmosphere at 30-900 ℃ and a heating rate of 20 ℃/min. The TG, DTA and DTG curves of PANI/SDS are shown in Fig.9. The TG of PANI/SDS shows that an initial mass loss (3.74%) between 50 and 128 ℃ is attributed to the loss of traces of water. The major mass losses are observed over two temperature periods. About 27.69% mass loss takes place in the temperature range between 128 and 320 ℃, and about 26.82% mass loss takes place in the temperature range between 320 and 565 ℃, respectively. Even at 900 ℃, the complex still maintains about 40% mass. DTA analyses reveal that melting of the sample with a pronounced exothermic effect at 385 ℃ takes place, which confirms that PANI/SDS complex catalytic synthesized by FeTSPc has strong thermal stability.
Fig.9 TG, DTA and DTG curves of PANI/SDS complex
4 Conclusions
(1) The synthesis of an electroactive PANI in the aqueous micelle solutions is accomplished using an environmentally friendly process. The biomimetic FeTSPc-catalyzed PANI synthesis is carried out with SDS to improve solubility and process ability of the conducting polymer.
(2) FeTSPc, as a mimetic enzyme for the synthesis of PANI, is inspired by its high activity, good stability, high solubility and extremely inexpensive cost. It enjoys distinct advantages over similar reactions catalyzed by native enzyme.
(3) The use of micelles as template in the mimetic enzymatic synthesis of conducting polyaniline may offer new possibility for tailoring the electrochemical and chemical properties of polyaniline.
References
[1] AKKARA J A, SENCEAL K J, KAPLAN D L. Synthesis and characterization of polymers produced by horseradish peroxodase in dioxane [J]. Journal of Polymer Science: Polymer Chemistry Edition, 1991, 29(3): 1561-1568.
[2] SAKHAROV I Y, VOROBIEV A K, CASTILLO LEON J J. Synthesis of polyelectrolyte complexes of polyaniline and sulfonated polystyrene by palm tree peroxidase [J]. Enzyme and Microbial Technology, 2003, 33(9): 661-667.
[3] KARAMYSHEV A V, SHLEEV S V, KOROLEVA O V, YAROPOLOV A I, SAKHAROV I Y. Laccase-catalyzed synthesis of conducting polyaniline [J]. Enzyme and Microbial Technology, 2003, 33(9): 556-564.
[4] SAKHAROV I Y, OUPOROV I V, VOROBIEV A K, ROIG M G, PLETJUSHKINA O Y. Modeling and characterization of polyelectrolyte complex of polyaniline and sulfonated polystyrene produced by palm tree peroxidase [J]. Synthetic Metals, 2004, 142(1/3): 127-135.
[5] HU X, ZHANG Y Y, TANG K, ZOU G L. Hemoglobin-biocatalysts synthesis of a conducting molecular complex of polyaniline and sulfonated polystyrene [J]. Synthetic Metals, 2005, 150(1): 1-7.
[6] HU X, ZHANG Y Y, LI C H, LIU H H, ZOU G L. Synthesis of a conducting polyaniline by hemoglobin as biocatalyst [J]. Acta Chimica Sinica, 2005, 63(1): 33-38. (in Chinese)
[7] HU X, SHU X S, LI X W, LIU S G, ZHANG Y Y, ZOU G L. Hemoglobin-biocatalyzed synthesis of conducting polyaniline in micellar solutions [J]. Enzyme and Microbial Technology, 2006, 38(5): 675-682.
[8] SAITO Y, MIFUNE M, NAKASHIMA S, ODO J. Determination of hydrogen peroxide with N,N-diethylaniline and 4-aminoantipyrine by use of an anion-exchange resin modified with manganese-tetrakis (sulphophenyl) porphine, as a substitute for peroxidase [J]. Talanta, 1987, 34: 667-669.
[9] GROOTBOOM N, NYOKONG T J. Iron perchlorophthalocyanine and tetrasulfophthalocyanine catalyzed oxidation of cyclohexane using hydrogen peroxide, chloroperoxybenzonic acid and tert-butylhydroperoxide as oxidants [J]. Journal of Molecular Catalysis A: Chemical, 2002, 179: 113-123.
[10] HANASAKI N, MATSUDA M, TAJIMA H, NAITO T, INABE T. Torque study of TPP[Fe(Pc)(CN)2]2 [J]. Synthetic Metals, 2003, 137: 1227-1228.
[11] ELLIS S, KOZHEVNIKOV I V. Homogeneous oxidation of methyl isobutyrate with oxygen catalysed by metal complexes: polyoxometalates versus metalloporphyrins and metallo- phthalocyanines [J]. Journal of Molecular Catalysis A: Chemical, 2002, 187: 227-235.
[12] MILOS M. A comparative study of biomimetic oxidation of oregano essential oil by H2O2 or KHSO5 catalyzed by Fe(Ⅲ) meso- tetraphenylporphyrin or Fe(Ⅲ) phthalocyianine [J]. Applied Catalysis A: General, 2001, 216: 157-161.
[13] HABER J, PAMIN K, POLTOWICZ J. Cationic metalloporphyrins and other macrocyclic compounds in zeolite matrix as catalysts for oxidation with dioxygen [J]. Journal of Molecular Catalysis A: Chemical, 2004, 224: 153-159.
[14] LIU W, CHOLLI A L, NAGARAJAN R, KUMAR J. TRIPATHY S K, SENECAL K J, BRUNO F F, SAMUELSON L A. The role of template in the enzymatic synthesis of conducting polyaniline [J]. Journal of American Chemical Society, 1999, 121: 11345-11355.
[15] KIM B J, OH S G, HAN M G, IM S S. Preparation of polyaniline nanoparticles in micellar solutions as polymerization medium [J]. Langmuir, 2000, 16: 5841-5845.
[16] WEBER J H, BUSCH D H. Complexes derived from strong field ligands XIX magnetic properties of transition metal derivatives of 4,4′,4″,4′″-tetrasulfo-phthalocyanine [J]. Inorganic Chemistry, 1965, 4(4): 469-471.
[17] WUDL F, ANGUS R O, LU F L, ALLEMAND P M, VACHON D J, NOWAK M, LIU Z X, HEEGER A J. Poly-phenyleneamineimine: Synthesis and comparison to polyaniline [J]. Journal of American Chemical Society, 1987, 109: 3677-3684.
[18] LIU W, KUMAR J, TRIPATHY S, SAMUELSON L A. Enzymatic synthesis of conducting polyaniline in micelle solutions [J]. Langmuir, 2002, 18: 9696-9704.
[19] LIU W, KUMAR J, TRIPATHY S K, SENECAL K J, SAMUELSON L A. Enzymatically synthesized conducting polyaniline [J]. Journal of American Chemical Society, 1999, 121: 71-78.
[20] PREMACHANDRAN R S, BANERJEE S, WU X K, JOHN V T, MCPHERSON G L, AKKARA J A, AYYAGARI M, KAPLAN D L. Enzymatic synthesis of fluorescent naphthol-based polymers [J]. Macromolecules, 1996, 29: 6452-6460.
[21] SAMUELSON L A, ANAGNOSTOPOULOS A, ALVA K S, KUMAR J, TRIPATHY S K. Biologically derived conducting and water soluble polyaniline [J]. Macromolecules, 1998, 31: 4376-4378.
[22] YUE J, WANG Z H, CROMACK K R, EPSTEIN A J, MACDIARMID A G. Effect of sulfonic acid group on polyaniline backbone [J]. Journal of American Chemical Society, 1991, 113: 2665-2671.
[23] KIM B J, OH S G, HAN M G, IM S S. Synthesis and characterization of polyaniline nanoparticles in SDS micellar solutions [J]. Synthetic Metals, 2001, 122: 297-304.
[24] BERRY B C, SHAIKH A U, VISWANATHAN T. Corrosion prevention of cold rolled steel using water dispersible lignosulfonic acid-doped polyaniline [J]. Polymer Preparation, 2000, 41: 1739- 1740.
[25] ROY S, FORTIER J M, NAGARAJAN R, TRIPATHY S K, KUMAR J, SAMUELSON L A, BRUNO F F. Biomimetic synthesis of a water soluble conducting molecular complex of polyaniline and lignosulfonate [J]. Biomacromolecules, 2002, 3: 937-941.
[26] VARELA H, DE ALBUQUERQUE MARANHAO S L, MELLO R M Q, TICIANELLI E A, TORESSI R M. Comparisons of charge compensation process in aqueous media of polyaniline and self-doped polyanilines [J].Synthetic Metals, 2001, 122: 321-327.
(Edited by CHEN Wei-ping)
Foundation item: Project(07JJ6020) supported by the Hunan Provincial Natural Science Foundation of China; Project(2007-24-3) supported by the Huaihua Key Science and Technology Program, China
Received date: 2008-12-26; Accepted date: 2009-03-12
Corresponding author: HU Xing, PhD; Tel: +86-745-2851037; E-mail: huxing152@sohu.com
- Iron(Ⅱ) tetrasulfophthalocyanine mimetic enzymatic synthesis ofconducting polyaniline in micellar system
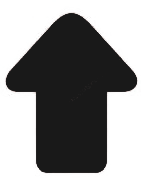