- Abstract:
- 1 Introduction▲
- 2 Experimental▲
- 3 Simulation▲
- 4 Conclusions▲
- References
- Figure
- Fig.1 Schematic diagram of experiment apparatus
- Fig.2 Solidification microstructures of d300 mm round ingot at different ultrasonic powers: (a) P=0 W; (b) P=240 W
- Fig.3 Solidification microstructures at ultrasonic power of 240 W and different casting speeds: (a) v=40 mm/min (Central part); (b) v=40 mm/min (Edge part); (c) v=45 mm/min (Central part); (d) v=45 mm/min (Edge part); (e) v=50 mm/min (Central part); (f) v= 50 mm/min (Edge part)
- Fig.4 Thermal conductivity of 7050 aluminum alloy
- Fig.5 Enthalpy of 7050 aluminum alloy
- Fig.6 Distribution of nucleation parameters
- Fig.7 Microstructure distribution of ingot at different ultrasonic powers: (a) 0 W; (b) 240 W
- Fig.8 Effect of ultrasonic power on ingot grain refinement
- Fig.9 Effect of casting speed on grain sizes in ultrasonic field
J. Cent. South Univ. Technol. (2010) 17: 431-436
DOI: 10.1007/s11771-010-0502-8
Effect of ultrasonic power and casting speed on solidification structure of
7050 aluminum alloy ingot in ultrasonic field
ZHANG Li-hua(张立华)1, 2, YU Jun(余军)1, 2, ZHANG Xiao-ming(张晓明)1, 2
1. Key Laboratory of Modern Complex Equipment Design and Extreme Manufacturing, Ministry of Education,
Central South University, Changsha 410083, China;
2. School of Mechanical and Electrical Engineering, Central South University, Changsha 410083, China
? Central South University Press and Springer-Verlag Berlin Heidelberg 2010
Abstract:
With the experiment and finite element simulation, the influences of power ultrasonic on the solidification structure of 7050 aluminum alloy ingot in semi-continuous casting were researched, and the effects of casting speed on solidification structure in ultrasonic field were also analyzed. The experiment and simulation results show that the solidification structure of the ingot is homogeneously distributed, and its grain size is obviously refined at ultrasonic power of 240 W. The average grain sizes, which can be seen from the Leica microscope, are less than 100 ?m. When the casting speed is 45-50 mm/min, the best grain refinement is obtained.
Key words:
1 Introduction
The high-alloy aluminum alloy 7050 is a light structural material that is widely used in aviation manufacturing. With the high-speed development of modern aeronautics and astronautics, many researchers in the world attach great importance to the preparing methods of high-alloy aluminum alloy. The process of casting is widely used in the preparation of aluminum products. Direct chill is a prevailing semi-continuous casting method in the production of aluminum alloy ingots [1-6]. The difficulties in the casting process of high-strength aluminum alloy are as follows: due to the spatial scale effects and non-uniform casting environment, the temperature field and melt flow field of the solidification region are unevenly distributed; the internal stress in large ingot is out of limit and the solidification structure distributes very irregularly in the space. The situation is more serious for the production of high-alloy aluminum ingot. The development of an advanced melting and casting technology for smelting homogeneous and flawless high-quality ingot is a prerequisite to produce large-scale and high-performance components. How to get homogeneous and fine grains, and how to suppress the initiation and growth of crack through the regulation of ultrasonic field in the casting process are always concerned by the scholars at home and abroad.
ESKIN [7-8], and PAN et al [9] began to study the fine-grained ultrasonic technology from the 20th century. Many experimental researches were also done by domestic scholars, such as LI and MOMONO [10], ZHAO and MU [11], and LI et al [12]. The results show that, the microstructure and mechanical properties of castings can be improved by the ultrasonic cavitation effect [13-14]. In order to suppress the cracking tendency of the large-scale and high-alloy aluminum ingot in the process of semi-continuous casting, the solidification process can be controlled by the imposition of ultrasonic field, and a reasonable technological regulation is expected [15]. After that, the grain size is refined, the segregation is reduced, and the initiation and growth of the crack are inhibited and prevented. In this work, the influences of casting parameters, such as ultrasonic power and casting speed, on the solidification structure of 7050 aluminum alloy ingot in semi- continuous casting were investigated.
2 Experimental
2.1 Schemes
After melting the high-purity aluminum, the middle alloy with a certain proportion was added one by one. The 7050 aluminum alloy was configured when it was melted. The ultrasonic generator was composed of a piezoelectric ceramic transducer (PZT), a ladder-shaped horn and a titanium instrument stroke. Its work-end diameter was 50 mm. The experimental ultrasonic power was 2 kW (adjustable) and its frequency was 20 kHz. The diameter of aluminum alloy ingot was 300 mm. The casting length was 2 m. The thermocouple was placed at 75 mm away from the edge of the mold in order to test the change of melt temperature. The initial melt depth was set up to 150 mm. The ultrasonic device was put on the top of the mold. The insertion depth of ultrasonic horn was 20 mm. The constant flow calorimetry method was used to calibrate the actual output power of the ultrasonic vibration, and the result was 240 W. Fig.1 shows the sketch map of experimental device.
Fig.1 Schematic diagram of experiment apparatus
At the beginning of the experiment, the thermocouple was used to test the pouring temperature in launder and melt temperature in crystallizer. The results were 680 and 660 ℃, respectively. The ultrasonic vibration was added into the melt after the ingot length reached about 350 mm. The casting speed was changed in the rest part of the experiment. Four 20 mm-thick cross-sections were chosen from d300 mm round ingot: one from the non-ultrasonic effect part, the other three from the ultrasonic effect part, by which the casting speeds were 40, 45 and 50 mm/min, respectively. Two small pieces were selected from the middle and edge parts from each separately cross-section to grind, do chemical polishing and corrosion. Leica microscope was used to observe its solidification microstructure.
2.2 Materials
ICP-AES method was used to detect the components of the experimental materials. The chemical composition is shown in Table 1. The components of alloy are accorded with the requirements of 7050 aluminum alloy.
Table 1 Chemical composition of 7050 aluminum alloy (mass fraction, %)
2.3 Results and discussion
The effect of ultrasonic vibration on the solidification microstructures of round ingot is shown in Fig.2. In the ultrasonic effect area, the impact of casting speed on solidification microstructures is shown in Fig.3.
Fig.2 Solidification microstructures of d300 mm round ingot at different ultrasonic powers: (a) P=0 W; (b) P=240 W
Fig.3 Solidification microstructures at ultrasonic power of 240 W and different casting speeds: (a) v=40 mm/min (Central part); (b) v=40 mm/min (Edge part); (c) v=45 mm/min (Central part); (d) v=45 mm/min (Edge part); (e) v=50 mm/min (Central part); (f) v= 50 mm/min (Edge part)
The internal and external microstructures of the round ingot are smaller under the effect of ultrasonic compared with the non-ultrasonic vibration part. The distributions of microstructures of the round ingot are more homogeneous (as shown in Figs.2 and 3) and the average grain size is less than 100 ?m. The grain refinement effect of ultrasonic power on the microstructures is indicated in the experimental results. The reason is probably that the dendritic crystals are broken by the ultrasonic cavitations. On the one hand, the fragmentation can effectively inhibit the formation of coarse grains; on the other hand, a large number of small broken dendritic crystals, which are produced by the fragmentation, become the new nuclei. As a result, the nucleation rate is improved and more small grains are formed. At the same time, the small broken dendrites spread to all parts of the cast ingot with the help of ultrasonic stirring. The nucleation of the ingot is promoted, and then more homogeneous microstructures are formed.
As can be seen from Table 2, with the increase of casting speed, the grain sizes of central ingot become smaller and those of the edge parts get larger. This phenomenon may be influenced by the liquid cave shape and the effect scope of ultrasonic field. As a result, with the increase in casting speed, the liquid cave becomes deeper and the liquid-solid interface will come down. Then the broken dendrites, which are produced by the ultrasonic cavitations, are away from the high- temperature melt (the sprue gate is located at the upper middle of the casting mold). The re-melting is prevented, which is propitious to the preservation of broken dendrites and the enhancement of nucleation. It is worth noticing that, as the effect scope of ultrasonic field in melt is limited, if the casting speed is too fast, the corresponding effect time by ultrasonic will be shortened. As a result, the effect of melt mixing is insufficient and the substantive nucleation and effective diffuse in the middle of the ingot are unable to form. This may lead to great difference in solidification structure between the center and edge parts of the ingot, even affect the grain refinement.
Table 2 Average grain sizes of ingot at different casting speeds
3 Simulation
3.1 Simulation parameters
As the symmetry of the model structure was taken into consideration in the model building, the simulation model was chosen from a quarter of the ingot and mould. The diameter of mould was 300 mm, and the height was 200 mm. The initial melt height was 100 mm. The melt surface was 20 mm under the top of the mold, and the upper surface of the dummy head was 80 mm above the bottom of the mold. The pouring temperature was set to 680 ℃. The first cooling water intensity was 500 W/(m2?K), and the second one was 2 kW/(m2?K). The casting speeds were 40, 45, 50 and 55 mm/min, respectively.
Some parameters of the 7050 aluminum alloy were: its Poisson ratio was 0.33; liquidus temperature was 635 ℃ and solidus temperature was 524 ℃. The remaining boundary conditions were set by the Mile algorithm, which was chosen from the semi-continuous casting module in software ProCAST. After putting the composition of alloy into the software, the thermal physical parameters of 7050 aluminum alloy were calculated from the thermodynamic database. Two of them are shown in Figs.4 and 5. It can be seen that the enthalpy of 7050 aluminum alloy increased with increasing temperature, but the thermal conductivity decreased when the temperature was 450-650 ℃ and increased at other temperatures. The change of enthalpy was used to dispose the release process of the solidification latent heat in the simulation process.
Fig.4 Thermal conductivity of 7050 aluminum alloy
Fig.5 Enthalpy of 7050 aluminum alloy
3.2 Simulation finite element theory
As the actual effect of ultrasonic cavitation on the solidification structure is hard to simulate at present, the following assumptions and treatments were used in the simulation process: (1) all of the cross-sections in the liquid cave area were affected by the ultrasonic, and the attenuation effect did not exist; (2) the ultrasonic power was included in the cavitation threshold of ultrasonic in the aluminum alloy melt; (3) the impact of grain refiner on the ingot structure was neglected; and (4) the stirring action of acoustic streaming on the aluminum alloy melt was ignored. The simulation research of solidification structures under ultrasonic field was implemented by modifying the nucleation distribution parameters in ProCAST. As shown in Fig.6, the calculation model of grain density was used to calculate the grain structure feature in the solidification process, and the Gaussian continuous nucleation model founded by Rappaz was taken as the grain nucleation model.
Fig.6 Distribution of nucleation parameters
The nucleation density of grain at a certain undercooling was calculated by [16]:
(1)
where ?T is the nucleation undercooling; n is the grain density; nmax is the initial nucleation parameter of primary dendrite phase, namely the maximum nucleation density of Gaussian distribution; ?Tσ is the secondary nucleation parameter of primary dendrite phase, namely the standard deviation of Gaussian distribution; ?Tn is the third nucleation parameter of primary dendrite phase, namely the average undercooling of Gaussian distribution. Eq.(2) can be obtained from Eq.(1):
(2)
The nucleation distribution parameters of aluminum alloy were obtained from the parameter database of ProCAST, and the nucleation density of different nucleation undercooling in ultrasonic field was measured in the experiment, as shown in Table 3.
Table 3 Grain density of 7050 aluminum alloy at different nucleation undercooling
An equation was obtained after putting the data in Table 3 into Eq.(2). As shown in Table 4, the nucleation distribution parameters ?Tn, nmax and ?Tσ of the aluminum alloy, which were formed in the ultrasonic field, were calculated after Eq.(2) was solved.
Table 4 Nucleation parameters of 7050 aluminum alloy
3.3 Simulation results and analysis
The simulation results show that, under the effect of power ultrasonic of 240 W, the inside and outside ingot microstructures become more homogeneous. The microstructure distribution of the ingot is shown in Fig.7.
Fig.7 Microstructure distribution of ingot at different ultrasonic powers: (a) 0 W; (b) 240 W
As can be seen from Fig.8, the average grain sizes of the ingot are between 800 and 1 700 ?m in the absence of ultrasonic. But the average sizes are between 80 and 115 ?m under the effect of power ultrasonic of 240 W. From the middle to the edge of the ingot, the grain size decreases. The sizes at the edge of the ingot are the smallest. This is because the nucleation of a melt is mainly affected by the supercooling degree. As the attenuation of ultrasonic field in the melt is neglected, the actual undercooling is more effective and the grain size is the smallest in edge part of the melt.
Fig.8 Effect of ultrasonic power on ingot grain refinement
The effect of casting speed in ultrasonic field on the grain size distribution of the ingot is shown in Fig.9. From Fig.9, it can be seen that when the casting speed is 45-50 mm/min (at this point the depth of liquid cave is about 192 mm), the grain sizes in central part of the ingot are much smaller. But when the speed is too slow (about 40 mm/min), the central ingot sizes are much larger. In the experimental part, the grain sizes in the central part are also smaller than those in the edge part when the casting speed is 45-50 mm/min (Table 2). This is influenced by the liquid cave shape and the affecting scope of ultrasonic field. The simulation results are basically consistent with the experimental results, showing the credibility of the simulation data.
Fig.9 Effect of casting speed on grain sizes in ultrasonic field
4 Conclusions
(1) The experimental results show that, the solidification structure of 7050 aluminum alloy melt is obviously refined and its distribution is more homogeneous after adding the ultrasonic field in the process of semi-continuous casting. The average grain sizes are less than 100 ?m by the imposition of ultrasonic field.
(2) By setting the impact of effective undercooling on the nucleation rate in ultrasonic field, the effect of ultrasonic on the solidification structure of the ingot is approximately simulated. With the comparison of the simulation and experiment results, the average grain size difference is less than 5 ?m. So the simulation results are creditable.
(3) The change of casting speed in ultrasonic field has a great influence on the homogeneity of the solidification structure. The experiment and simulation results prove that when the casting speed is 45- 50 mm/min, that is, when the liquid cave depth is about 192 mm, the ultrasonic grain refinement is the best and the ingot solidification structure is the most uniform.
References
[1] ZHANG Lei, SHEN Hou-fa, RONG YI-ming, HUAN Tian-you. Numerical simulation on solidification and thermal stress of continuous casting billet in mold based on meshless methods [J]. Materials Science and Engineering A, 2007, 466(1/2): 71-78.
[2] LE Qi-chi, GUO Shi-jie, ZHAO Zhi-hao, CUI Jian-zhong, ZHANG Xin-jian. Numerical simulation of electromagnetic DC casting of magnesium alloys [J]. Journal of Materials Processing Technology, 2007, 183(2/3): 194-201.
[3] ZHANG H T, NAGAUMIB H, YUBO Z, CUI J Z. Coupled modeling of electromagnetic field, fluid flow, heat transfer and solidification during low frequency electromagnetic casting of 7XXX aluminum alloys: PartⅠ. Development of a mathematical model and comparison with experimental results [J]. Materials Science and Engineering A, 2007, 448(1/2): 189-203.
[4] ZHANG H T, NAGAUMIB H, CUI J Z. Coupled modeling of electromagnetic field, fluid flow, heat transfer and solidification during low frequency electromagnetic casting of 7XXX aluminum alloys: Part Ⅱ. The effects of electromagnetic parameters on casting processes [J]. Materials Science and Engineering A, 2007, 448(1/2): 177-188.
[5] VERTNIKA R, ZALOZNIKB M, SARLER B. Solution of transient direct-chill aluminum billet casting problem with simultaneous material and interphase moving boundaries by a meshless method [J]. Engineering Analysis with Boundary Elements, 2006, 30(10): 847-855.
[6] DU Q, ESKIN D G, KATGERMAN L. The effect of ramping casting speed and casting temperature on temperature distribution and melt flow patterns in the sump of a DC cast billet [J]. Materials Science and Engineering A, 2005, 413/414: 144-150.
[7] ESKIN G I. Ultrasonic treatment of molten aluminum [M]. Moscow: Metallurgiya, 1985: 1-10.
[8] ESKIN G I. Ultrasonic treatment of light alloy melts [M]. Amsterdam: Gordon & Breach, 1998: 1-5.
[9] PAN J, YOSHIDA M, SASAKI G. Ultrasonic insert casting of aluminum alloy [J]. Scripta Materialia, 2000, 43(2): 155-159.
[10] LI J W, MOMONO T. Effect of starting temperature of ultrasonic application on the structure of Al-Cu alloy ingots [J]. Foundry Technology, 2004, 25(4): 248-251.
[11] ZHAO Zhong-xing, MU Guang-hua. Effect of ultrasonic on the structure and properties of casting alloy [J]. Foundry, 1996, 18(3): 21-25. (in Chinese)
[12] LI Ying-long, LI Bao-mian, LIU Yong-tao, GAO Cai-ru, DAI En-tai. Effects of high-intensity ultrasonic on structures and properties of Al-Si alloys [J]. The Chinese Journal of Nonferrous Metals, 1999, 9(4): 719-722. (in Chinese)
[13] LI J W, MOMONO T, FU Y, JIA Z, TAYU Y. Effect of ultrasonic stirring on temperature distribution and grain refinement in Al-1.65%Si alloy melt [J]. Transactions of Nonferrous Metals Society of China, 2007, 17(4): 691-697.
[14] GAO Xue-peng, LI Xin-tao, QIE Xi-wang, WU Ya-ping, LI Xi-meng, LI Ting-ju. Effect of ultrasonic field on the structures and mechanical properties of horizontal continuous casting ingots of Al-1%Si alloy [J]. Acta Metallurgica Sinica, 2007, 43(1): 17-22. (in Chinese)
[15] HU Shi-cheng, MA Wei-ce, DU Lei. Thermal contact conductance at continuous roll-casting interface [J]. Journal of Central South University of Technology, 2007, 14(3): 374-379.
[16] RAPPAZ M, GANDIN C A. Probabilistic modeling of microstructure formation in solidification processes [J]. Acta Metall, 1993, 41(2): 345-360.
Foundation item: Project(2010CB731700) supported by the National Basic Research Program of China
Received date: 2009-06-30; Accepted date: 2009-10-09
Corresponding author: ZHANG Li-hua, PhD, Associate professor; Tel: +86-731-88876167; E-mail:zhanglihua_csu@163.com
- Effect of ultrasonic power and casting speed on solidification structure of7050 aluminum alloy ingot in ultrasonic field
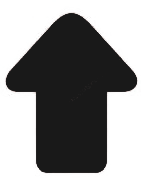