- Abstract:
- 1 Introduction▲
- 2 Model▲
- 3 Simulation▲
- 4 Conclusions▲
- References
- Figure
- Fig.1 Relationships between equilibrium speed and density (a), and between equilibrium flow and density (b)
- Fig.2 Evolutions of uniform flow (Δh= 0.7 m): (a) ρ0=0.04 veh/m; (b) ρ0= 0.05 veh/m; (c) ρ0=0.06 veh/m; (d) ρ0= 0.07 veh/m; (e) ρ0=0.08 veh/m
- Fig.3 Evolutions of uniform flow (Δh= -0.7 m): (a) ρ0=0.04 veh/m; (b) ρ0= 0.05 veh/m; (c) ρ0=0.06 veh/m; (d) ρ0= 0.07 veh/m; (e) ρ0=0.08 veh/m
- Fig.4 Evolutions of small perturbation (Δh=0.7 m): (a) ρ0=0.03 veh/m; (b) ρ0= 0.04 veh/m; (c) ρ0=0.05 veh/m; (d) ρ0=0.06 veh/m; (e) ρ0=0.07 veh/m; (f) ρ0=0.08 veh/m
- Fig.5 Evolutions of small perturbation (Δh=-0.7 m): (a) ρ0=0.03 veh/m; (b) ρ0= 0.04 veh/m; (c) ρ0=0.05 veh/m; (d) ρ0=0.06 veh/m; (e) ρ0=0.07 veh/m; (f) ρ0=0.08 veh/m
J. Cent. South Univ. Technol. (2011) 18: 1757-1764
DOI: 10.1007/s11771-011-0899-8
Macro modeling and analysis of traffic flow with road width
TANG Tie-qiao(唐铁桥)1, 2, LI Chuan-yao(李传耀)1, HUANG Hai-jun(黄海军)2, SHANG Hua-yan(尚华艳)3
1. School of Transportation Science and Engineering,Beijing University of Aeronautics and Astronautics, Beijing 100191, China;
2. School of Economics and Management, Beijing University of Aeronautics and Astronautics, Beijing 100191, China;
3. Information College, Capital University of Economics and Business, Beijing 100070, China
? Central South University Press and Springer-Verlag Berlin Heidelberg 2011
Abstract:
The macro modeling and the solution of traffic flow with road width were investigated. Firstly, a new macro model with the consideration of road width was proposed. Secondly, the effects of road width on uniform flow and small perturbation were studied. The analytical and numerical results show that widening (shrinking) road can enhance (reduce) the equilibrium speed and flow, and the increments (decrements) will increase with the absolute value of road width gradient. In addition, the numerical results illustrate that the new model can describe the effects of road width on the evolutions of uniform flow and small perturbation.
Key words:
traffic flow; road width; macro modeling; perturbation;
1 Introduction
Various traffic problems have become more and more serious, which has attracted scholars to develop many traffic flow models to describe various complex traffic phenomena [1]. LIGHTHILL and WHITHAM [2] and RICHARDS [3] independently proposed the first macro model (called as LWR model), CHEN et al [4] later presented a high-resolution relaxation scheme and ZHANG and SHU [5] recently developed a finite volume scheme with a high order accuracy. The LWR model can reproduce the formation, propagation and evolution of shock, but it does not describe the non-equilibrium traffic flow because the speed cannot be deviated from the equilibrium speed. To overcome this shortcoming, scholars later proposed some density-gradient (DG) models [6-7] and speed-gradient (SG) ones [8-15]. The first DG model was developed by PAYNE [6]. This model can describe some non-equilibrium traffic phenomena but it cannot be adopted to directly study mixed traffic flow. Thus, GUPTA and KATIYAR [7] proposed another DG model for heterogeneous traffic flow. However, these DG models will produce backward movement under some specific conditions since they have a characteristic speed greater than the speed of vehicle. In order to conquer this drawback, JIANG et al [8] presented a SG model and found that the drawback movement will not appear, but this model has some shortcomings, too. In order to further improve the model, HUANG et al [9] developed a new SG model for two-lane traffic flow and PENG et al [10] extended this work with the consideration of the coupling effects with lanes. TANG et al extended the model to describe the network flow [11], the heterogeneous flow [12], the impacts of ramps and the driver’s forecast effects on traffic flow [13-14]. YU and SHI [15] proposed a new anisotropic continuum model to investigate the traffic density wave. However, the above models could not be used to directly study the effects of road structure on traffic flow since they did not explicitly take this factor into account. In order to describe these effects, MICHALOPOULOS et al [16] considered the road geometry and developed a new macro model with the friction effects of on-off-ramps. WU [17] presented a fluid dynamic model with the road width based on the characteristics of the Chinese traffic. FENG [18] proposed a dynamic model with the road area to study the effects of the changeable area on traffic flow. TSAI and CHU [19] used case study to analyze the optimal investment in the length of road expansion. ZHANG and SHEN [20] carried out a numerical investigation to study the effects of the freeway lane drop on the driving behavior and found that the lane-drop site and its geometry may affect traffic flow. MATHEW and RADHAKRISHNAN [21] presented a new method to study the non-lane-based driving behaviors and developed a micro simulation model for the non-lane- based heterogeneous flow at the signalized intersections. JIN et al [22] proposed a non-lane-based full speed difference model to study the effects of road width on the car-following behavior. However, the above models could not completely describe the effects of road width on traffic flow because the gradient of road width is not explicitly considered at all. The purpose of this work is to present a new macro model to investigate the effects of road width on traffic flow and provide important information for designing the road width, the lane width and the number of lanes.
2 Model
The first macro model for traffic flow was a first-order continuum model (namely the LWR model in literature), i.e.
(1)
where t, x, ρ and ve(ρ) are the time, position, density and equilibrium speed, respectively. Equation (1) can reproduce the formation of shock, but it does not describe the non-equilibrium traffic flow because the speed cannot be deviated from the equilibrium speed ve(ρ). Later, many high order models were proposed to study the non-equilibrium traffic flow. The existing high order models can be divided into the density-gradient (DG) models and speed-gradient (SG) models, where the first DG model was the PAYNE model [6]:
(2)
where v is the speed; τ is the relaxation time; -0.5v′e(ρ), is the anticipation coefficient. And the simplest SG model was developed by JIANG et al [8]:
(3)
where c0 is the propagation speed of small perturbation.
The above models can describe some complex traffic phenomena, but they cannot be used to directly study the effects of road structure on traffic flow. In order to describe these effects, some extended models were developed [16-22], but they cannot describe the effects of road width on traffic flow since the gradient of road width is not explicitly considered. In the real traffic system, road is widened or shrank at some special positions and driver will accelerate when road is widened and decelerate when road is shrank, so the gradient of road width should explicitly be considered when studying the traffic flow. Thus, a new macro model can be obtained:
(4)
where A and σ are the road width and the reactive time that it takes driver to adjust his acceleration based on the
road width, respectively. is the adjustment term
of the road width. Note that σ is less than τ and |dA/dσ| should be less than σ/τ. Equation (4) explicitly considers the gradient of road width, so it can be used to study some complex phenomena resulted from the variable road width.
3 Simulation
3.1 Effects of road width on traffic flow
Set ρ0 and v0 as the equilibrium solutions and substitute them into Eq.(4). Equation (4) can be rewritten as
(5)
From Eq.(5), the equilibrium speed v0 and the equilibrium flow q0 can be obtained:
(6)
(7)
From Eq.(6) and Eq.(7), an important proposition can be obtained.
Proposition 1: Widening road width can enhance the equilibrium speed and flow and their increments will increase with the gradient of road width; shrinking road width will reduce the equilibrium speed and flow and their decrements will increase with the absolute value of the road width gradient.
In order to further describe the quantitative effects of road width on the equilibrium speed and flow, the equilibrium speed should be defined. The equilibrium speed and parameters have little qualitative effect on the following results, so the equilibrium speed can be defined as [23]
(8)
where vf is the free-flow speed and ρj is the jam density. And other parameters are: vf=20 m/s, ρj=0.2 veh/m, τ= 10 s, σ=5 s.
Applying the above parameters, the speed-density and flow-density curves could be obtained (see Fig.1). From Fig.1, the following conclusions could be drawn:
1) Since drivers often think that widening road width can make them accelerate and that the amplitude of their acceleration will increase with the gradient of road width, widening road width can enhance the equilibrium speed and flow and their increments will increase with dA/dx. Drivers often consider that shrinking road width can make them decelerate and that the amplitude of their deceleration will increase with the absolute value of the gradient of road width, so shrinking road width will reduce the equilibrium speed and flow and their decrements will increase with |dA/dx| (see Fig.1).
Fig.1 Relationships between equilibrium speed and density (a), and between equilibrium flow and density (b)
2) There is enough room for drivers to accelerate (decelerate) since the headway is relatively large whenthe density is relatively low, thus widening (shrinking) road width will have prominent effects on the equilibrium speed. There is little room for drivers to accelerate (decelerate) since the headway is relatively small when the density is relatively high, so widening (shrinking) road width will have little effect on the equilibrium speed. The room for drivers to accelerate (decelerate) decreases with the density, so the effects of widening (shrinking) road width on the equilibrium speed will turn weak (see Fig.1(a)).
3) When the density is very low or high,
ρ0ve(ρ0)-ρ0ve(ρ0) will be very small since ρ0ve(ρ0) is very small even though the equilibrium speed is prominently enhanced or reduced. When the density is moderate,
will be very prominent
since ρ0ve(ρ0) is relatively high even if the equilibrium
speed is slightly enhanced or reduced.
ρ0ve(ρ0)-ρ0ve(ρ0) will increase then decrease with the density. Thus, road width has little effect on the equilibrium flow when the density is very low or high and has great effects on the equilibrium flow when the density is moderate, and these effects first increase then decrease with density (see Fig.1(b)). These results just show that widening road width will enhance the road capacity while shrinking road width will reduce the road capacity.
3.2 Effects of road width on evolution of uniform flow
ZHANG et al [24-25] pointed out that the conservative scheme should be adopted to carry out numerical tests if using dynamics model to describe traffic flow, so Eq.(4) should be rewritten as the conservative form:
(9)
where
Thus, Eq.(9) can be approximated by the following scheme:
(10)
where i, n, Δx and Δt are the road section, the time interval, the space step and the time step, respectively.
In addition, can be defined as
(11)
where α=Δx/Δt(n) (Δt(n) is the time step at the n-th step). For simplicity, α is here set as vf (vf is the free-flow speed).
In the real traffic system, the road width and its gradient are very complex. For simplicity, the parameter of the road width is set as
(12)
where h0 is the mean road width; Δh is a small perturbation of the road width; x0 and Δ are two parameters. In the numerical tests, the road is set as one ring whose length is 32.2 km. Other parameters are as follows:
(13)
Using the above parameters, the evolutions of uniform flow under different perturbations of road width are shown in Fig.2 and Fig.3. From these two figures, the following conclusions could be obtained.
1) When the initial density is relatively low or high, the small perturbation of the road width has little effect on uniform flow, i.e. the traffic flow is approximately uniform flow and slight change appears only near the small perturbation because the traffic flow is stable at this time (see Fig.2(a), 2(e), 3(a) and 3(e)). This shows that the effects of the small perturbation of the road width on uniform flow will quickly be dissipated when the initial density is relatively low or high.
Fig.2 Evolutions of uniform flow (Δh= 0.7 m): (a) ρ0=0.04 veh/m; (b) ρ0= 0.05 veh/m; (c) ρ0=0.06 veh/m; (d) ρ0= 0.07 veh/m; (e) ρ0=0.08 veh/m
Fig.3 Evolutions of uniform flow (Δh= -0.7 m): (a) ρ0=0.04 veh/m; (b) ρ0= 0.05 veh/m; (c) ρ0=0.06 veh/m; (d) ρ0= 0.07 veh/m; (e) ρ0=0.08 veh/m
2) When the initial density is moderate, the small perturbation of the road width will destroy uniform flow and produce stop-and-go since uniform flow is easily destroyed at this time (see Figs.2(b)-(d) and Figs.3(b)- (d)). What’s more, the stop-and-go will become first more serious then slighter with the increase of the initial density. These results show that road width will have great effects on uniform flow when density is moderate.
3.3 Effects of road width on evolution of small perturbation
For simplicity, the following perturbation is adopted [26]:
(14)
where L is the road length. Other parameters are the same as those in Fig.2 and Fig.3. The evolutions of Eq.(14) under the small perturbation of the road width are shown in Fig.4 and Fig.5. From these figures, the following results could be obtained.
1) The small perturbation will quickly evolve into uniform flow when the initial density is relatively low or high, since traffic flow is stable at this time (see Figs.4(a), 4(f), 5(a) and 5(f)) and immediately produce stop-and-go when 0.03 veh/m<ρ0<0.08 veh/m because traffic flow is unstable at this time (see Figs.4(b)-4(e) and Figs.5(b)- 5(e)).
2) Compared with Fig.2, Fig.3 and the results in Ref.[8], the down critical value is reduced and the stop-and-go is more serious, which shows that the small perturbation of the density and the small perturbation of the road width will both reduce the stability of traffic flow.
3) There is little prominent difference between Fig.4 and Fig.5, but their results show that widening or shrinking the road width will both destroy the stability of traffic flow and make stop-and-go more serious.
Fig.4 Evolutions of small perturbation (Δh=0.7 m): (a) ρ0=0.03 veh/m; (b) ρ0= 0.04 veh/m; (c) ρ0=0.05 veh/m; (d) ρ0=0.06 veh/m; (e) ρ0=0.07 veh/m; (f) ρ0=0.08 veh/m
4) The above results show that the abrupt change should be avoided when designing the road width, the lane width or the number of lanes since the stability of traffic flow may be destroyed when the density is moderate.
By comparing with Fig.2-Fig.5, the following results could be obtained. When the initial density is moderate and the same, the stop-and-go in Fig.3 is slightly more serious than that in Fig.2 and the stop-and-go in Fig.5 is slightly more serious than that in Fig.4, which show that, when the road width produces small perturbation, shrinking road width will have more negative effects on traffic flow than widening road width.
4 Conclusions
1) A new macro model with the consideration of road width is developed based on the effects of road width on traffic flow. This model can describe the effects of road width on the traffic flow and reproduce some complex phenomena resulted from the road width.
2) There is a positive relation between road width and the equilibrium speed and flow.
3) The effects of small perturbation of road width on the uniform flow are investigated. Small perturbation of road width will destroy the uniform flow when the initial density is moderate and has little effect on the uniform flow when the initial density is very low or very high.
4) Small perturbations of the road width and density will both destroy the stability of traffic flow and make stop-and-go more serious when the initial density is moderate and have little effect on the uniform flow when the initial density is very low or very high.
Fig.5 Evolutions of small perturbation (Δh=-0.7 m): (a) ρ0=0.03 veh/m; (b) ρ0= 0.04 veh/m; (c) ρ0=0.05 veh/m; (d) ρ0=0.06 veh/m; (e) ρ0=0.07 veh/m; (f) ρ0=0.08 veh/m
References
[1] CHOWDHURY D, SANTEN L, SCHADSCHNEIDER A. Statistical physics of vehicular traffic and some related systems [J]. Physical Report, 2000, 329: 199-329.
[2] LIGHTHILL M J, WHITHAM G B. On kinematic waves: II. A theory of traffic flow on long crowed roads [C]// Proceeding Royal Society London, 1955, 229: 317-345.
[3] RICHARDS P I. Shock waves on the highway [J]. Operational Research, 1956, 4: 42-51.
[4] CHEN Jian-zhong, SHI Zhong-ke, HU Yan-mei. A relaxation scheme for multi-class Lighthill: Whitham-Richards traffic flow model [J]. Journal of Zhejiang University: Science A, 2009, 10: 1835-1844.
[5] ZHANG Xiang-xiong, SHU Chi-wang. A genuinely high order total variation diminishing scheme for one-dimensional scalar conservation laws [J]. SIAM Journal on Numerical Analysis, 2010, 48: 772-975.
[6] PAYNE H J. Models of freeway traffic and control [C]// Mathematical Models of Public Systems. La Jolla: Simulation Councils Proceedings Series, 1971: 51-61.
[7] GUPTA A K, KATIYAR V K. A new multi-class continuum model for traffic flow [J]. Transportmetrica, 2007, 3: 73-85.
[8] JIANG Rui, WU Qing-song, ZHU Zuo-jin. A new continuum model for traffic flow and numerical tests [J]. Transportation Research B, 2002, 36: 405-419.
[9] HUANG Hai-jun, TANG Tie-qiao, GAO Zi-you. Continuum modeling for two-lane traffic flow [J]. Acta Mechanica Sinica, 2006, 22: 131-137.
[10] PENG Guang-han, SUN Di-hua, He Heng-pan. A new traffic model with the consideration of coupling effect with lanes [J]. Chinese Physics B, 2009, 18: 468-474.
[11] TANG Tie-qiao, HUANG Hai-jun, MEI Chao-qun, ZHAO Shou-gen. A dynamic model for traffic network flow [J]. Physica A, 2008, 387: 2803-2810.
[12] TANG Tie-qiao, HUANG Hai-jun, SHANG Hua-yan. A new dynamic model for heterogeneous traffic flow [J]. Physics Letter A, 2009, 373: 2461-2466.
[13] TANG Tie-qiao, HUANG Hai-jun, WONG S C, GAO Zi-you, ZHANG Ying. A new macro model for traffic flow on a highway with ramps and numerical tests [J]. Communications in Theoretical Physics, 2009, 51: 71-78.
[14] TANG Tie-qiao, HUANG Hai-jun, SHANG Hua-yan. A new macro model for traffic flow with the consideration of the driver’s forecast effect [J]. Physics Letter A, 2010, 374: 1668-1672.
[15] YU Lei, SHI Zhong-ke. Density wave in a new anisotropic continuum model for traffic flow [J]. International Journal of Modern Physics C, 2009, 20: 1849-1859.
[16] MICHALOPOULOS P G, YI P, LYRINTZIS A S. Development of an improved high order continuum traffic flow model [J]. Transportation Research B, 1993, 27: 125-132.
[17] WU Zheng. A fluid dynamics model for low speed traffic system [J]. Acta Mechanic Sinica, 1994, 26: 149-157. (in Chinese)
[18] FENG Su-wei. Mathematical modeling, field calibration and numerical simulation of low-speed mixed traffic flow in cities [D]. Shanghai: Shanghai University, 1997. (in Chinese)
[19] TSAI J F, CHU C P. Analysis of the optimal length of road expansion-A case study of the Taipei metropolitan area [J]. Transportation Research A, 2010, 44: 147-158.
[20] ZHANG H M, SHEN Wei. Numerical investigation of stop-and-go traffic patterns upstream of freeway lane drop [J]. Transportation Research Record, 2009, 2124: 3-17.
[21] MATHEW T V, RADHAKRISHNAN P. Calibration of micro simulation models for non-lane-based heterogeneous traffic at signalized intersections [J]. Journal of Urban Planning and Development-ASCE, 2010, 136: 59-66.
[22] JIN Sheng, WANG Dian-hai, TAO Peng-fei, LI Ping-fan. Non-lane-based full velocity difference car-following model [J]. Physica A, 2010, 389: 4654-4662.
[23] KERNER B S, KONH?USTER P. Cluster effect in initially homogeneous traffic flow [J]. Physical Review E, 1993, 48: R2335-R2338.
[24] ZHANG Peng, WONG S C, DAI Shi-qiang. Characteristic parameters of a wide cluster in a higher-order traffic flow model [J]. Chinese Physics Letters, 2006, 23: 516-519.
[25] ZHANG Peng, WONG S C. Essence of conservation forms in the traveling wave solutions of higher-order traffic flow models [J]. Physical Review E, 2006, 74: 026109.
[26] HERMANN M, KERNER B S. Local cluster effect in different traffic flow models [J]. Physica A, 1998, 255: 163-188.
(Edited by YANG Bing)
Foundation item: Project(NCET-08-0038) supported by the Program for New Century Excellent Talents in Chinese University; Projects(70701002, 70971007 and 70521001) supported by the National Natural Science Foundation of China; Project(2006CB705503) supported by the National Basic Research Program of China
Received date: 2010-08-31; Accepted date: 2011-01-08
Corresponding author: TANG Tie-qiao, Associate Professor, PhD; Tel: +86-10-82339327; E-mail: tieqiaotang@buaa.edu.cn
- Macro modeling and analysis of traffic flow with road width
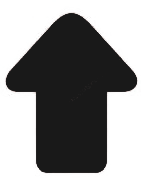