- Abstract:
- 1 Introduction▲
- 2 Experimental▲
- 2.1 Microorganisms
- 2.2 Culture media and growth conditions
- 2.3 Assay of antagonistic activity
- 2.4 Time course of antagonistic activity of BS501a FFS
- 2.5 Optimization of physical parameters of fermentation and metal inorganic salts in medium using one-factor-at-a-time method
- 2.6 Optimization of physical parameters of fermentation and metal inorganic salts in medium using orthogonal array
- 3 Results and discussion▲
- 3.1 Time course of antagonistic activity of BS501a FFS
- 3.2 Optimization of physical parameters of fermentation using one-factor-at-a-time
- 3.3 Optimization of physical parameters of fermentation by orthogonal array method
- 3.4 Optimization of metal inorganic salts using one- factor-at-a-time
- 3.5 Optimization of metal inorganic salts by orthogonal array method
- 4 Conclusions▲
- References
- Figure
- Fig.1 Time course of antagonistic activity of B. subtilis BS501a FFS
- Fig.2 Effect of initial pH value on antagonistic activity of B. subtilis BS501a FFS
- Fig.3 Effect of temperature on antagonistic activity of B. subtilis BS501a FFS
- Fig.4 Effect of metal inorganic salts in medium on antagonistic activity of B. subtilis BS501a FFS
J. Cent. South Univ. Technol. (2011) 18: 1047-1053
DOI: 10.1007/s11771-011-0802-7
Fermentation optimization to improve production of antagonistic metabolites by Bacillus subtilis strain BS501a
LI Rui-fang(李瑞芳), XU Yi(徐怡)
College of Bioengineering, Henan University of Technology, Zhengzhou 450001, China
? Central South University Press and Springer-Verlag Berlin Heidelberg 2011
Abstract:
To improve antagonistic metabolites production of Bacillus subtilis strain BS501a, physical parameters of fermentation and metal inorganic salts in medium, namely initial pH value, culture temperature, fermentation time, concentrations of CaCl2, FeSO4, ZnSO4, MnSO4 and MgSO4, were optimized using one-factor-at-a-time and orthogonal tests. The results show that the optimal physical parameters of fermentation are an initial pH of 7.0, a culture temperature of 30 °C, and a fermentation time of 48 h. The optimal concentrations of metal inorganic salts in basal medium are 10.2 mmol/L CaCl2, 0.4 mmol/L FeSO4, 3.5 mmol/L ZnSO4, 0.6 mmol/L MnSO4 and 2.0 mmol/L MgSO4. Among the metal inorganic salts, MgSO4 and MnSO4 play important roles in the improvement of the antagonistic metabolites production of B. subtilis strain BS501a; especially, MgSO4 contributes a highly significant effect. The average diameter of inhibition zone of the BS501a filtered fermentation supernatant (FFS) cultured in the optimal fermentation conditions against Magnaporthe grisea DWBJ329 reaches 71.4 mm, and there is 2.4-fold increase in antifungal activity as compared with 21.2 mm under the pre-optimized conditions.
Key words:
Bacillus subtilis; antagonistic metabolites; production; optimization; orthogonal array;
1 Introduction
Rice blast is a destructive disease caused by Magnaporthe grisea (T. T. Hebert) Barr (anamorph= Pyricularia grisea (Cooke) Sacc.) that seriously limits rice production in rice growing regions. The disease mainly occurs as leaf or neck blast. Although the neck blast has a greater impact on yield, the leaf blast can cause severe damage before plants reach the reproductive growth stage.
In recent years, natural methods of growing crops have attracted much attention in the context of a trend towards agriculturally and environmentally sustainable development [1]. Microbe has been regarded as a good bioresource for contribution to resolving the recent world problems, such as food and energy crises and environmental pollution, and for production of high added value compounds for medicine and pharmacy [2]. The first successful biocontrol agent used against insects and pathogens was a member of the genus, Bacillus [3]. One beneficial rhizobacterium, B. subtilis, a ubiquitous bacterium in soil that promotes plant growth and protects plants from fungal pathogen attacks [4-5], shows antagonistic activities against several plant pathogens. Till now, several Bacillus subtilis strains and their derivatives are found to have broad suppressive abilities over a variety of plant pathogens in vitro [6-8] by producing antagonistic metabolites, such as lipopeptide antibiotics iturin A [9] and biosurfactin [10].
Different environmental factors and medium components affect the metabolites of Bacillus subtilis. There are some literatures on fermentation optimization of Bacillus subtilis [9-11], with more attention paid to carbon and nitrogen sources, but less attention on minerals except MgSO4, K2HPO4 and KH2PO4. There are many metal ions in soil, such as K+, Ca2+, Na+, Mg2+, Zn2+, Fe2+, Fe3+, Mn2+, Co2+, Ni2+ and Cu2+. The metabolites production of B. subtilis will be affected by these metal ions without a doubt. B. subtilis BS501a, with antagonistic activity to Magnaporthe grisea, is a strain isolated from the rhizosphere of rice. In the present study, orthogonal array (Taguchi methodology) is used to optimize the physical parameters of fermentation (temperature, initial pH, fermentation time), and metal inorganic salts (such as CaCl2, FeSO4, MnSO4, MgSO4, ZnSO4) to improve the antagonistic metabolites production of B. subtilis BS501a in shake flask and investigate their interactions.
2 Experimental
2.1 Microorganisms
B. subtilis strain BS501a (GenBank accession No. FJ755787) was screened from the rhizosphere of rice 10 cm below the surface of the soil in the rice-growing area near the Yellow River in Zhengzhou, Henan Province, China. Magnaporthe grisea DWBJ329 was kindly provided by Dr. LIANG Shen (Zhejiang University, China).
2.2 Culture media and growth conditions
Luria–Bertani (LB) medium (peptone 1%, yeast extract 0.5%, NaCl 1%, pH 7.2) was used for inoculums preparation, with the addition of 20 g/L agar for isolate maintenance.
The basal medium used for antagonistic metabolites production contained the following constituents (g/L): sucrose 30, peptone 10, yeast extract 5, K2 HPO4 3, and pH value was 7.0.
The seed culture was prepared by transferring a loop of B. subtilis BS501a cells from a fresh culture grown onto LB agar into 25 mL LB broth in 250 mL Erlenmeyer flask. The flask was incubated at 220 r/min and 37 °C for 16 h.
The production process was carried out in Erlenmeyer flasks (250 mL) containing 50 mL aliquots of the fermentation medium under test. The flasks were inoculated with the seed culture at 5% (volume fraction) and incubated in a shaking incubator (220 r/min). B. subtilis strain BS501a was fermented in the flasks containing the basal medium for 48 h at 37 °C in physical parameter optimization tests and 48 h at 30 °C in metal inorganic salts optimization tests. The culture medium was centrifuged at 1×104g for 20 min and the filtered fermentation supernatant (FFS) was obtained after the fermentation supernatant filtered with 0.22 μm filter paper to analyze the antagonistic activity against M. grisea.
2.3 Assay of antagonistic activity
Antagonistic activity was determined by well diffusion assays. A spore suspension (100 μL) of M. gresea (approximately 109 cfu/mL) was spread onto potato dextrose agar (PDA) plates. The plates were then aseptically punched with 6 mm holes in the agar using a cork borer and 100 μL of the BS501a FFS was added to the wells. For each plate tested, one well was filled with the solvent (fresh culture medium) as a negative control. The plates were then left undisturbed to allow the solvent to diffuse into the agar and then were incubated at 28 °C for 4 d. Diameters of inhibition zone were measured [12]. All experiments were carried out in triplicate and the mean values were reported with standard error.
2.4 Time course of antagonistic activity of BS501a FFS
The time course of antagonistic activity of B. subtilis BS501a FFS in basal medium was studied to determine the time range in one-factor-at-a-time tests and the maximum antagonistic activity of BS501a FFS in basal medium. The fermentation was maintained for 60 h. The diameters of inhibition zone of B. subtilis BS501a FFS at time interval of 4 h were investigated. The experiment was replicated three times.
2.5 Optimization of physical parameters of fermentation and metal inorganic salts in medium using one-factor-at-a-time method
The one-factor-at-a-time method was used to investigate the effects of environmental factors including temperature, pH value and concentrations of metal inorganic salts such as CaCl2, FeSO4, ZnSO4, MnSO4 and MgSO4. It involved changing one independent variable while fixing all the others at a certain level.
2.5.1 Effect of initial pH
In order to investigate the effect of initial pH of fermentation medium on the antagonistic metabolites production of B. subtilis BS501a, the seed culture of B. subtilis BS501a was cultivated in basal medium with different initial pH values (5.0-8.5) and fermented in shake flask. The antagonistic activity of BS501a FFS was determined by well diffusion assays.
2.5.2 Effect of temperature
In order to investigate the effect of temperature on the antagonistic metabolites production of B. subtilis BS501a, the seed culture of B. subtilis BS501a was cultivated in basal medium and fermented at different temperatures (28-37 °C) in shake flask. The antagonistic activity of BS501a FFS was determined by well diffusion assays.
2.5.3 Effects of metal inorganic salts in medium
The effects of different metal inorganic salts on antagonistic metabolites production of B. subtilis BS501a were studied by adding them into the basal medium respectively at different concentrations, i.e., CaCl2·2H2O 0.5-3 g/L, FeSO4·7H2O 0.05-2.0 g/L, ZnSO4·7H2O 0.05-2.0 g/L, MnSO4·H2O 0.05-2.0 g/L, MgSO4·7H2O 0.1-2.0 g/L. The seed culture of B. subtilis BS501a was cultivated in the tested medium and fermented in shake flask. The antagonistic activity of BS501a FFS was determined by well diffusion assays.
2.6 Optimization of physical parameters of fermentation and metal inorganic salts in medium using orthogonal array
To get the maximum yield of antagonistic metabolites and identify significant variables, orthogonal array (Taguchi design) was carried out to examine the interactions and optimize the variables of fermentation. Experimental designs and analysis of experimental data were performed by using software Orthogonal Experimental Assistant 3.1. The variables for first design were initial pH, temperature and fermentation time, and those for second were CaCl2.2H2O, FeSO4?7H2O, ZnSO4?7H2O, MnSO4?H2O and MgSO4?7H2O.
3 Results and discussion
3.1 Time course of antagonistic activity of BS501a FFS
The antagonistic activity profile of BS501a FFS in basal medium (Fig.1) shows that a fermentation time of 48 h is required for the maximum production of antagonistic metabolites. The antagonistic activity increases from 8 to 48 h during fermentation, and then remains almost constant after 48 h. The maximum diameter of the inhibition zone is 21.2 mm. Some literatures reported that the maximum antagonistic metabolites production by B. subtilis S3 [9] and B. subtilis BS5 [10] was at 7 d, and by B. subtilis B02 it was at 72 h [13]. Strain BS501a produces the maximum antagonistic metabolites in shorter time.
Fig.1 Time course of antagonistic activity of B. subtilis BS501a FFS
3.2 Optimization of physical parameters of fermentation using one-factor-at-a-time
3.2.1 Optimization of initial pH of fermentation medium
Figure 2 shows the effects of different initial pH value of fermentation medium on the antagonistic activity of BS501a FFS. An initial pH value of 7.0-7.2 supports the maximum production of antagonistic metabolites. The pH value of B. subtilis required for the maximum antagonistic metabolites production is about 7 from Ref.[13] and 6.0-6.8 from Refs.[9-10]. From the results of this investigation and Refs.[9-10, 13], the fermentation time that B. subtilis strains produce the maximum antagonistic metabolites is shorter in neutral environment than in a slightly acidic environment.
Fig.2 Effect of initial pH value on antagonistic activity of B. subtilis BS501a FFS
3.2.2 Effect of temperature
Figure 3 shows that 30 °C supports the maximum production of antagonistic metabolites. The result is similar to the results in other literatures [9-10, 13]. Figure 3 also shows that the change of temperature has little effect on the antagonistic activity of BS501a FFS. Perhaps the antagonistic compounds in BS501a FFS are heat-resistant.
Fig.3 Effect of temperature on antagonistic activity of B. subtilis BS501a FFS
3.3 Optimization of physical parameters of fermentation by orthogonal array method
An L9 orthogonal matrix was used to investigate the significant factors of fermentation conditions obtained from one-factor-at-a-time (Table 1).
The results of the orthogonal experiments are shown in Table 2, in which K1j , K2j , and K3j are the averages of the responses corresponding to levels 1, 2, and 3, respectively, and R=max(Kij)-min(Kij). The larger the value of Kij, the better the condition is, and the larger the value of R, the greater effect the factor has on the antagonistic metabolites production of BS501a. On the base of this idea, according to the values of Kij in Table 2, the order in which the individual parameter affects on the antifungal metabolites production of BS501a is temperature > initial pH value > fermentation time, suggesting that temperature has the maximum effect and fermentation time has the least effect on the antifungal metabolites production of BS501a. Compared with the result of one-factor-at-a-time test, the change of temperature has effect on the antagonistic metabolites production of BS501a when the initial pH value and the fermentation time are changed.
Table 1 Levels and their values of physical parameters of fermentation for orthogonal experiments
Table 2 Antagonistic activity of BS501a FFS in orthogonal experiments for physical parameters of fermentation
On the other hand, when the initial pH value, temperature and fermentation time are at level 2, B. subtilis BS501a has the maximum antagonistic metabolites production. These levels also represent the optimum physical parameters of fermentation.
In variance analysis, F0.05(2, 2)=19.00 is taken as a reference value, and F>19.00 means a significant effect of that factor on response. According to the results of variance analysis in Table 3, factors as initial pH, temperature and fermentation time whose F values are less than 19.00, have insignificant effect on antagonistic metabolites production of BS501a.
Table 3 Results of response variance analysis
It can be concluded that the optimal parameters of fermentation for the antagonistic metabolites production of BS501a are as follows: initial pH value 7.0, temperature 30 °C, and fermentation time 48 h. The effects of initial pH, temperature and fermentation time on the antagonistic metabolites production of BS501a are insignificant.
Under these conditions, i.e., 30 °C, pH 7.0 and 48 h, when B. subtilis BS501a cells are fermented in basal medium, a maximum diameter of inhibition zone, 26.3 mm of FFS, is obtained. Compared with the diameter of inhibition zone, 21.2 mm of BS501a, cultured in basal medium at pre-optimization, a 0.2-fold increase is obtained.
3.4 Optimization of metal inorganic salts using one- factor-at-a-time
Figure 4 shows that metal inorganic salts have important influence on the production of antagonistic metabolites of BS501a, especially MgSO4 and MnSO4, and 1.5-2.0 g/L CaCl2?2H2O, 0.5 g/L FeSO4?7H2O, 1.0 g/L ZnSO4?7H2O, 0.1 g/L MnSO4?H2O and 0.5 g/L MgSO4?7H2O are required for the maximum antagonistic metabolites production.
3.5 Optimization of metal inorganic salts by orthogonal array method
An L16 orthogonal matrix was used to investigate the most significant factor of metal inorganic salts obtained from one-factor-at-a-time (Table 4).
The result of orthogonal experiment is listed in Table 5. According to the values of Kij in Table 5, the order that the individual metal ion affects the antagonistic metabolites production of BS501a is Mg2+>Mn2+>Ca2+>Fe2+>Zn2+, suggesting that Mg2+ has the maximum effect and Zn2+ has the least effect on antifungal metabolites production of BS501a.
On the other hand, the antagonistic activity of BS501a FFS is the maximum when Fe2+ is at level 1, Ca2+ is at level 2, Mg2+ and Mn2+ are at level 3, whereas Zn2+ is at level 4. These levels also represent the optimum concentrations of individual components in the medium.
Fig.4 Effect of metal inorganic salts in medium on antagonistic activity of B. subtilis BS501a FFS
Table 4 Levels and their values of metal inorganic salts in medium for orthogonal experiments
Table 5 Antagonistic activity of BS501a FFS in orthogonal experiments for metal inorganic salts
In the variance analysis, F0.05(3, 3)=9.280 and F0.01(3, 3)=29.500 are taken as the reference values, and F>9.280 means significant effect of that factor, F>29.500 means highly significant effect of that factor. According to the results of variance analysis in Table 6, MgSO4?7H2O whose F value is greater than 29.500, has highly significant effect on the antagonistic metabolites production, and MnSO4?H2O, whose F value is greater than 9.280, has significant effect on the antagonistic metabolites production, while other factors have insignificant effects.
Table 6 Results of response variance analysis
It can be concluded that the optimal concentration of metal inorganic salts for the highest antagonistic metabolites production are as follows: CaCl2?2H2O 1.5 g/L (10.2 mmol/L), FeSO4?7H2O 0.1 g/L (0.4 mmol/L), ZnSO4?7H2O 1.0 g/L (3.5 mmol/L), MnSO4?H2O 0.1 g/L (0.6 mmol/L) and MgSO4?7H2O 0.5 g/L (2.0 mmol/L).
Under the optimal fermentation conditions (30 °C, pH 7 and 48 h), B. subtilis BS501a is cultivated in the optimal fermentation medium (30 g/L sucrose, 10 g/L peptone, 5 g/L yeast extract, 3 g/L K2HPO4, 10.2 mmol/L CaCl2, 0.4 mmol/L FeSO4, 3.5 mmol/L ZnSO4, 0.6 mmol/L MnSO4, and 2.0 mmol/L MgSO4), and a maximum diameter of inhibition zone, 71.4 mm of B. subtilis BS501a FFS, is obtained. Compared with the diameter of inhibition zone, 21.2 mm of BS501a FFS, in the basal medium under pre-optimization condition, a 2.4-fold increase is obtained.
There are reports on the biological control of Bacillus subtilis on cucumber and red pepper fungal disease [6-7]. The inhibition zones of the better candidate strains are about 20 mm, but to the best of our knowledge, no reports are available on the investigation of the biological control of Bacillus subtilis to rice blast. After the optimization of the fermentation conditions, the biomass and antagonistic activity of Bacillus subtilis are improved. Compared with the results of 30-40 mm inhibition zone diameter reported in Ref.[13], the inhibition zone diameter of 71.4 mm obtained in this work is interesting. The results also show that inorganic ions in the medium play an important role in promoting the production of antagonistic secondary metabolites of Bacillus subtilis.
Biological control agents compete with difficulty against the chemically synthesized compounds on the market because of their high production cost [10]. While the strong efficacy of iturin A (one component of antifungal metabolites of B. subtilis) against various phytopathogenic fungi is similar to the available chemical pesticides [9], and the surfactin (another component of antifungal metabolites of B. subtilis) is a good dispersant and emulsifier for spraying application of B. subtilis FFS. B. subtilis is still recognized as a promising alternative of synthetic chemicals to control plant diseases [14-15]. Optimization of the fermentation condition to improve the antagonistic metabolites production of B. subtilis is an economic consideration of the production process.
Compared with other reports about product medium optimization [16-18], more metal inorganic salts are paid attention on. The effects of some metal inorganic salts generally existing in soil are investigated. Mg2+ is a “key” metal ion for the production of metabolites by Bacillus species without having effect on cell growth [10]. The results of this work validate this. Meanwhile, the results show that Mn2+ also has significant influence on the production of antagonistic metabolites by Bacillus species.
4 Conclusions
1) A combination of factors and their levels involved in the production of antagonistic metabolites by Bacillus subtilis BS501a is identified for obtaining the maximum yield. The effects of MgSO4 and MnSO4 are found to be significant, in which MgSO4 is highly significant, while the rest of the factors such as initial pH, temperature, fermentation time, CaCl2, FeSO4 and ZnSO4 are determined to be insignificant in the antagonistic metabolites production of Bacillus subtilis BS501a. The experimental design using the orthogonal methodology for optimizing the yield of antagonistic metabolites by BS501a is an efficient strategy.
2) The diameter of inhibition zone of B. subtilis BS501a FFS obtained from the optimized conditions reaches 71.4 mm, a 2.4-fold increase than that obtained from pre-optimization conditions.
References
[1] STURZ A V, CHRISTIE B R, NOWAK J. Bacterial endophytes: Potential role in developing sustainable systems of crop production [J]. Critical Reviews in Plant Sciences, 2000, 19(1): 1-30.
[2] NIE Zhen-yuan, XIA Jin-lan, LEVERT J M. Methodology of factorial design deriving guidelines for simulation of growth curve and production of sugars by Spirulina (Arthrospira) maxima [J]. Journal of Central South University of Technology, 2001, 8(4): 228-233.
[3] POWELL K A, JUSTUM A R. Technical and commercial aspects of biological control products [J]. Pesticide Science, 1993, 37(2): 315-321.
[4] ASAKA O, SHODA M. Biocontrol of Rhizoctonia solani damping- off of tomato with Bacillus subtilis RB14 [J]. Applied and Environmental Microbiology, 1996, 62(11): 4081-4085.
[5] UTKHEDE R S, SMITH E M. Promotion of apple tree growth and fruit production by the EBW-4 strain of Bacillus subtilis in apple replant disease soil [J]. Canadian Journal of Microbiology, 1992, 8(12): 1270-1273.
[6] CHUNG S, KONG H, BUYER J S, LAKSHMAN D K, LYDON J, KIM S D, ROBERTS D P. Isolation and partial characterization of Bacillus subtilis ME488 for suppression of soilborne pathogens of cucumber and pepper [J]. Applied Microbiology and Biotechnology, 2008, 80(1): 115-123.
[7] LEE K J, KAMALA-KANNAN S, SUB H S, SEONG C K, LEE G W. Biological control of Phytophthora blight in red pepper (Capsicum annuum L.) using Bacillus subtilis [J]. World Journal of Microbiology and Biotechnology, 2008, 24(7): 1139-1145.
[8] SINGH N, PANDEY P, DUBEY R C, MAHESHWARI D K. Biological control of root rot fungus Macrophomina phaseolina and growth enhancement of Pinus roxburghii (Sarg.) by rhizosphere competent Bacillus subtilis BN1 [J]. World Journal of Microbiology and Biotechnology, 2008, 24(9): 1669-1679.
[9] SHIH Ing-lung, LIN Chih-yang, WU Jane-yii, HSIEH Chienyan. Production of antifungal lipopeptide from Bacillus subtilis in submerged fermentation using shake flask and fermentor [J]. Korean Journal of Chemical Engineering, 2009, 26(6): 1652-1661.
[10] ABDEL-MAWGOUD A M, ABOULWAFA M M, HASSOUNA N A-H. Optimization of surfactin production by Bacillus subtilis isolate BS5 [J]. Applied Biochemistry and Biotechnology, 2008, 150(1): 305--325.
[11] CAI Cheng-gang, ZHENG Xiao-dong. Medium optimization for keratinase production in hair substrate by a new Bacillus subtilis KD-N2 using response surface methodology [J]. Journal of Industrial Microbiology Biotechnology, 2009, 36(7): 875-883.
[12] XU Feng, MENG Kun, WANG Ya-ru, LUO Hui-ying, YANG Pei-long, WU Ning-feng, FAN Yun-liu, YAO Bin. Eukaryotic expression and antimicrobial spectrum determination of the peptide tachyplesin II [J]. Protein Expression and Purification, 2008, 58(2): 175-183.
[13] DING Cui-zhen, QIU Ji-yan, LIU Wei-cheng, WU Yun-feng, ZHAO Da. Optimization of medial components and cultural conditions for improving antagonistic activity of Bacillus subtilis B02 [J]. Chinese Journal of Biological Control, 2008, 24(2): 159-163. (in Chinese).
[14] SHODA M. Bacterial control of plant diseases [J]. Journal of Bioscience and Bioengineering, 2000, 89(6): 515-521.
[15] GNANAMANICKAM S S. Biological control of crop diseases [M]. New York: Marcel Dekker Inc, 2002: 421-442.
[16] XIA Jin-lan, MENG Hao, WANG Run-min, ZHANG Cheng-gui, XIONG Jing, NIE Zhen-yuan, QIU Guan-zhou. Identification and fermentation optimization of protopectinase-overproducing strain Aspergillus niger CD-01 for pectin production [J]. Journal of Central South University of Technology, 2009, 16(1): 53-60.
[17] CHAUHAN A K, SURVASE S A, KISHENKUMAR J, ANNAPURE U S. Medium optimization by orthogonal array and response surface methodology for cholesterol oxidase production by Streptomyces lavendulae NCIM 2499 [J]. The Journal of General and Applied Microbiology, 2009, 55(3): 171-180.
[18] NAGARJUN P A, RAO R S, RAJESHAM S, RAO L V. Optimization of lactic acid production in SSF by Lactobacillus amylovorus NRRL B-4542 using Taguchi methodology [J]. Journal of Microbiology, 2005, 43(1): 38-43.
(Edited by YANG Bing)
Foundation item: Project(2010A210003) supported by Henan Province Natural Sciences Research Plan; Project(0910SGYS34370-2) supported by Zhengzhou City Science and Technology Research Plan; Project supported by the Youth Backbone Teacher of Universities in Henan Province Grants Plan
Received date: 2010-03-30; Accepted date: 2010-12-30
Corresponding author: LI Rui-fang, Associate Professor, PhD; Tel: +86-371-67756873; E-mail: lrf@haut.edu.cn
- Fermentation optimization to improve production of antagonistic metabolites by Bacillus subtilis strain BS501a
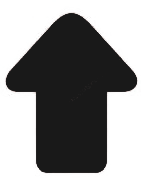