Flotation separation of chalcopyrite from galena by sodium humate and ammonium persulfate
Rui-zeng LIU1, Wen-qing QIN1, Fen JIAO1, Xing-jie WANG1, Bin PEI2, Yong-jun YANG2, Chun-hua LAI3
1. School of Minerals Processing and Bioengineering, Central South University, Changsha 410083, China;
2. Barun Mining Company, Inner Mongolia Baotou Steel Union Co., Ltd., Baotou 014080, China;
3. Zhengyuan Mining Co., Ltd., Honghe 662400, China
Received 3 March 2015; accepted 15 June 2015
Abstract: The flotabilities of chalcopyrite and galena with sodium humate (HA) and ammonium persulfate (APS) as the depressant were studied by flotation test, adsorption measurement and infrared spectroscopic analysis. Single mineral flotation test shows that the slurry oxidation environment and the proper oxidation of galena surface are prerequisites for the depression of galena by sodium humate. The closed-circuit flotation test of copper/lead bulk concentrate shows that the grade and recovery of Cu reach 30.47% and 89.16% respectively and those of Pb reach 2.06% and1.58% respectively in copper concentrate, and the grade and recovery of Pb reach 50.34% and 98.42% and those of Cu reach 1.45% and 10.84% respectively in lead concentrate with HA and APS. The selective depression effect of HA and APS is more obvious than that of potassium dichromate. The results of FTIR analysis and adsorption measurements indicate that the adsorption of sodium humate on the fresh surface of galena is negligible, while after oxidation, sodium humate can be chemically adsorbed on the surface of galena. According to the theory of solubility product, the sodium humate can display the oxidation product PbSO4, after then, adsorb on the surface of lead chemically to produce inhibitory effect. Thus, it can be seen that the combination of HA and APS is an efficient non-toxic reagent to achieve cleaning separation copper/lead bulk concentrate by flotation. The combination of HA and APS is an efficient non-toxic reagent to achieve cleaning for copper/lead bulk concentrate by flotation.
Key words: chalcopyrite; galena; sodium humate; sodium persulfate; flotation
1 Introduction
Chalcopyrite always coexists with other sulphide minerals, such as zinc and lead sulphide minerals [1]. Generally, two kinds of basic methods are commonly used for the separation of copper/lead minerals [2,3], such as copper depression with lead flotation and lead depression with copper flotation. Some reagents such as sodium cyanide for the depression of chalcopyrite and sodium dichromate for the depression of galena are employed [4]. Although only small amount of this kind of reagents were used, they caused serious environmental pollution [5]. So, some other environmentally friendly methods of depression are preferred.
According to the recent studies, the organic depressants have attracted a considerable amount of attention. In the laboratory and commercial processes, dextrin [6], starch [7], CMC [8] and ferrochrome lignosulfonate (FCLS) [9] have been used in the separation of chalcopyrite and galena. These kinds of reagents are nontoxic, biodegradable and relatively inexpensive compared with the widely used inorganic depressants.
Sodium humate is the sodium salt of humic acid and can be extracted from leonardite which is complicated, naturally forming organic compounds with high relative molecular mass. CHEN et al [10] indicate that sodium humate can be used as pyrite depressant in the separation of chalcopyrite and pyrite at lower pH values. The studies on the flotation behavior of pyrite and arsenopyrite in the xanthate and sodium humate systems showed that the sodium humate can depress arsenopyrite well [11,12].
There have been some studies on the separation of actual copper/lead ore with sodium humate as the depressant of galena [12,13], while the mechanism is still unclear and the depressing effect is uncertain. The aim of this work is to determine the depression mechanism of galena with sodium humate as the inhibitor, and put forward an environmentally friendly way for the separation of copper/lead minerals. The floatabilities of chalcopyrite and galena in the presence and absence of the depressants were studied. The adsorption measurement and infrared spectroscopic analysis were conducted to investigate the interaction between reagents and minerals.
2 Experimental
2.1 Materials and reagents
Galena and chalcopyrite samples were obtained from Bainiuchang Mine in Yunnan Province, China. The samples were purified by hand sorting, and the X-ray powder diffraction data confirmed the high purity of the samples. The chemical analysis revealed that the galena content (mass fraction) of the sample was 96.5% and the chalcopyrite content was 94.9%. The samples were respectively dry-ground in a porcelain ball mill and dry- screened to obtain particles with size range of 38-74 μm for the single mineral flotation and adsorption measurements. The fraction of particles with size larger than 74 μm was reground to the desired size fraction. A small part of particles with size less than 38 μm was further comminuted using agate mortar to 2 μm for infrared spectroscopic analysis, whereas the fraction of particles ranging in 38-74 μm was used. The ground products were stored separately in sealed glass bottles with nitrogen protection against further surface oxidation.
The sample of copper/lead bulk concentrate was obtained from the mineral processing plant of Bainiuchang, and the chemical compositions (mass fraction) are 9.60% Cu and 36.76% Pb.
Industrial grade butyl xanthate (BX, purity>85%) and methyl isobutyl carbinol (MIBC) were respectively used as the collector and frother, which were provided by Zhuzhou Flotation Reagents Factory in Hunan Province, China. Chemical grade sodium humate and sodium persulfate were obtained from Tianjin Guangfu Fine Chemical Research Institute (Tianjin, China). The solutions of all the reagents were prepared using distilled water. The dilute solutions of sodium hydroxide and hydrochloric acid were used as pH modifier.
2.2 Flotation tests
Flotation tests of single minerals were carried out in the micro-flotation cell with an effective volume of about 40 mL and the flowsheet is shown in Fig. 1. A 2.0 g mineral sample (38-74 μm size fraction) was used in each experiment and was cleaned ultrasonically prior to the tests. After agitation for 1 min, the pH of the pulp was firstly adjusted to a desired value by adding pH modifier. Then, the ammonium persulfate (APS) and/or (HA) sodium humate solution (adjust to neutral pH by H2SO4 in order to avoid the pH fluctuation) was added to the cell. Five minutes later, the samples were conditioned with xanthate for 2 min. After that, MIBC was added to the slurry. The flotation time was set to 2 min in all of the single-mineral experiments.
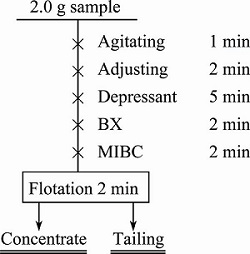
Fig. 1 Flowsheet of flotation tests on single minerals
The closed-circuit flotation test of copper/lead bulk concentrate was achieved in an XFG single-trough flotation machine with effective volumes of 0.25, 0.5, 0.75 and 1.0 L. The flowsheet is shown in Fig. 2.
2.3 Adsorption tests
The adsorption of HA on chalcopyrite and galena both in the absence and presence of different concentrations of APS at pH=6.5 was determined by measuring the residual concentration with total organic carbon analyzer. SHIMADZU total organic carbon analyzer of Japanese (TOC-L) was used in the test.
2.0 g mineral sample of 38-74 μm size fraction was cleaned by ultrasonic and then repulped with 50 mL distilled water at desired pH. After addition of the desired reagents, the solution was agitated with the identical condition as described in flotation test. Then, the pulp was centrifuged by centrifugation at 9000 r/min for 10 min using a high speed refrigerated centrifuge. The supernatants were withdrawn for the analysis of residual HA concentrations. The adsorption amount was calculated from the difference between initial and residual concentrations of the solution.
2.4 FTIR studies
The infrared spectra of galena, HA, the oxidized product of galena by APS, and the interaction products of unoxidized/oxidized galena with HA were obtained using Shimadzu IR Affinity-1 spectrometer.
Samples with size of 2 μm were prepared in the same way used in micro-flotation tests. 1.0 g sample was settled in 20 mL reagent solution at pH 6.5 and agitated for 30 min, filtrated and flushed 3 times with the corresponding pH buffer solution. The treated sample was dried in a vacuum desiccator for infrared spectrometry.
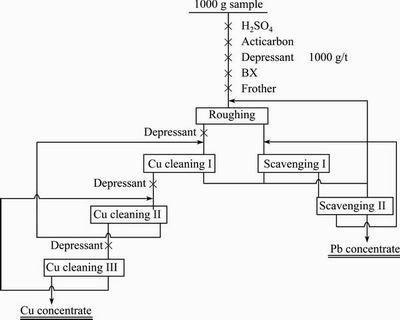
Fig. 2 Flowsheet of closed-circuit flotation test of copper/lead bulk concentrate
3 Results and discussion
3.1 Flotation tests of single mineral
3.1.1 Flotation of chalcopyrite and galena under different pH conditions without depressant
In this experiment, the pH of the pulp was adjusted with NaOH or HCl, and the BX and MIBC were used as the collector and frother, respectively. No depressant was added. The relationship between mineral floatability and pH value was determined (see Fig. 3). The resultsindicate that chalcopyrite and galena are easy-floating minerals with BX as collector in the whole pH ranges. However, the floatability of galena decreases rapidly when the pH value is higher than 9.
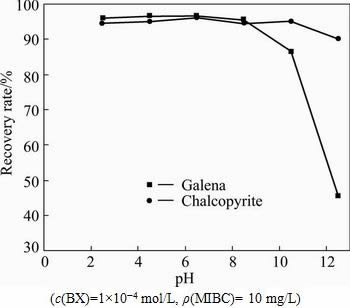
Fig. 3 Effects of pH value on recovery rate of chalcopyrite and galena with BX as collector
3.1.2 Flotation of chalcopyrite and galena with HA as depressant
The effects of HA on the flotation recoveries of chalcopyrite and galena at pH 6.5 were determined (see Fig. 4). The results indicate that with the increase of HA concentration from 0 to 75 mg/L, the floatabilities of the both minerals remained relatively constant, while as the concentration increases higher than 75 mg/L, the floatabilities decrease rapidly. However, the floatability difference between chalcopyrite and galena is very small, so it is impossible to achieve the separation purpose of copper/lead minerals.
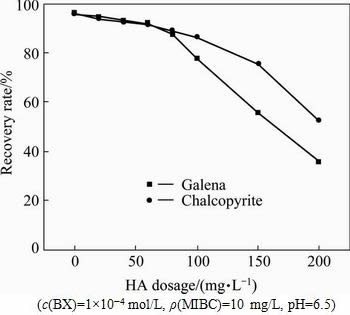
Fig. 4 Effects of HA dosage on recovery rates of chalcopyrite and galena with BX as collector
Several investigations [12,13] have confirmed that HA was a selective depressant of galena, but in those studies, redox conditions of the slurry were not taken into consideration.
3.1.3 Flotation of chalcopyrite and galena with APS as depressant
Sodium persulfate is often used as a strong oxidant. In the separation of copper/lead minerals by flotation, APS can be used as the depressant of galena. The effects of APS on the recovery rates of chalcopyrite and galena at pulp pH=6.5 were determined (see Fig. 5). The results indicate that with the increase of APS concentration from 0 to 200 mg/L, the floatabilities of both minerals remain relatively constant. It is impossible to achieve the separation purpose of copper/lead minerals by increasing the concentration of APS.
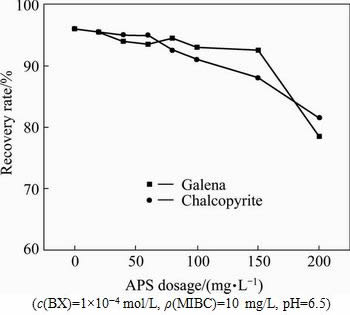
Fig. 5 Effects of APS dosage on recovery rates of chalcopyrite and galena with BX as collector
3.1.4 Flotation of chalcopyrite and galena with HA+APS as depressant
The addition of single depressant did not reach the purpose of the selective separation of copper/lead minerals. So, HA and APS were used together to study their synergistic effects on the separation of copper/lead minerals. The pulp pH was adjusted to 6.5 with HCl, and then HA and APS were added by the mass ratio of 1:1 and held for 5 min. The results (see Fig. 6) indicate that in the full range of the reagent concentration studied, with the increasing dosage of depressants, the floatability of chalcopyrite decreases slightly, while the floatability of galena decreases sharply. When the reagent concentration was above 30 mg/L (m(HA):m(APS)=1:1), the galena was hardly floatable, while the floatability of chalcopyrite remains relatively constant. With the synergistic effect of HA and APS, the floatability differences of the two minerals were enough to achieve the purpose of copper/lead separation.
In order to find out the mechanism of the synergistic effect, in the following studies, APS concentration remains constant at 5 mg/L and HA concentration gradually increases. With the increasing dosage of HA, the floatability of galena firstly decreases slightly, and then remains relatively constant in the range of 5-30 mg/L (see Fig. 7). The results indicate that the proper oxidation of galena is a prerequisite for the depression of galena by HA.
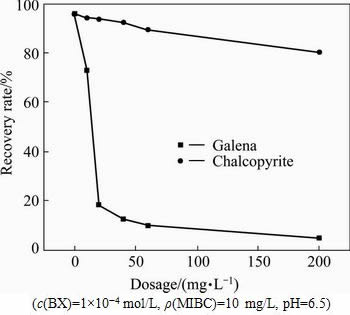
Fig. 6 Effects of HA+APS (mass ration, 1:1) dosage on recovery rates of chalcopyrite and galena with BX as collector
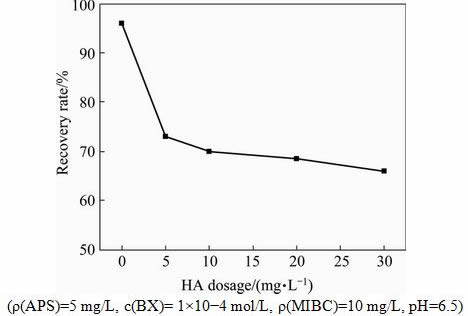
Fig. 7 Effects of HA dosage on recovery rates of chalcopyrite and galena with BX as collector
3.1.5 Separation of artificial mixed minerals
Chalcopyrite and galena were mixed in mass ratio of 1:2, and chemical compositions were 10.87% Cu and 55.72% Pb. 3 g mixed minerals were used in each experiment. HCl was added to obtain the desired pulp pH value of 6.5 and then the slurry was treated in the following order (see Fig. 1): depressant (HA 30 mg/L, APS 50 mg/L), collector (BX 2×10-5 mol/L) and frother (MIBC 10 mg/L). The flotation time was set to 2 min. The products and tailing were weighted separately after filtration and drying, and then analyzed for copper and lead separation (see Table 1).
Table 1 Results of copper/lead separation test of artificial mixed minerals by HA+APS
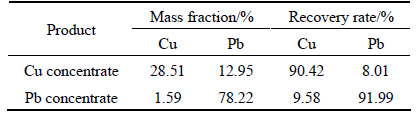
3.2 Closed-circuit flotation test in laboratory
The copper/lead bulk concentrate obtained from Bainiuchang Mineral Processing Plant, China, was tested in closed-circuit in site to avoid the change of mineral floatability in transit. Two schemes were employed by contrast test: 1) Depression of lead by sodium dichromate; 2) Depression of lead by HA+APS. H2SO4 was used to adjust the pulp pH, in the first scheme, pH=7.5 and in the second scheme, pH=6.5. The flotation flowsheet and reagent regime are shown in Fig. 2, and the results are shown in Table 2. The results illustrate that the recovery rates of chalcopyrite obtained by the two separation schemes have little difference. However, a decrease of the Pb content and increase of the Cu content in the corresponding concentrates were observed. It is because that galena in the bulk concentrate was selectively depressed in the presence of sodium humate [10].
Table 2 Results of copper/lead separation test of artificial mixed minerals by HA+APS
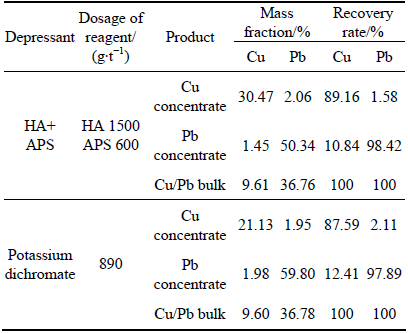
3.3 Flotation mechanism discussion
The aforementioned flotation tests indicate that the usage of single depressant (HA or APS) could hardly separate chalcopyrite and galena, but the mixed depressant (HA+APS) was highly selective in the separation of chalcopyrite and galena, and the proper oxidation of galena surface was a prerequisite for the depression of galena by HA. In this work, the solubility analysis, adsorption tests and the IR spectra were introduced to investigate their interactions.
Figure 8 illustrates the adsorption amount of HA on galena and chalcopyrite at different HA concentrations in the absence of APS. The results indicate that the adsorption of HA on galena and chalcopyrite increases slightly as the concentration of HA increases. The adsorption amount of HA on the galena is higher than that on the chalcopyrite.
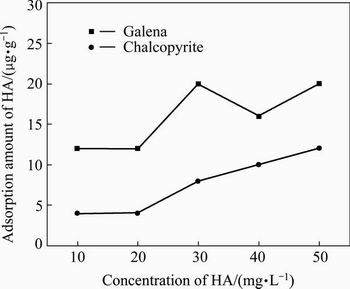
Fig. 8 Adsorption of HA onto chalcopyrite and galena at different HA concentrations and pH=6.5
Figure 9 illustrates the adsorption amount of HA on galena and chalcopyrite at different APS concentrations. The adsorption amount of HA on galena increases substantially as the concentration of APS increases. While, the adsorption of HA on chalcopyrite remains relatively constant and is independent of the APS concentration.
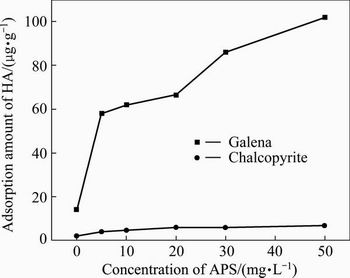
Fig. 9 Effects of APS concentration on adsorption of HA on chalcopyrite and galena with 20 mg/L HA, pH=6.5
The results of the absorption measurement agree well with the flotation test, and it is confirmed that the slurry oxidation environment is essential for the depression of galena by HA.
Table 3 lists the negative logarithm of the solubility product constant (Ksp) of the main lead compounds [14]. ZHUANG et al [15] had tested the apparent stability constant (Ks) of lead-humic acid complexes. When the pH is 6.5, the Ks is 10.65-12.3. The Ksp of PbS is 27.5, which is far greater than the Ks of PbnHA2. However, the Ksp of PbSO4 is less than the Ks of Pbn(HA)2. According to Taggart’s chemical reaction hypothesis [16], HA tends to react with PbSO4, which is the oxidation product of galena, to form Pbn(HA)2, while it can hardly react with PbS.
Table 3 Negative logarithm of solubility product constant (Ksp) of main lead compounds
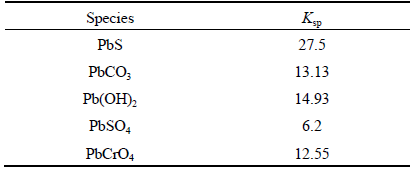
Infrared spectroscopy [17] was used to identify the surface species resulting from interactions between the galena and HA both before and after being oxidized by APS. The infrared spectrum shows only the 600-4000 cm-1 region, since this area includes most of the characteristic bands of HA. Figure 10 shows the infrared spectra of HA. It is illustrated that spectra have stretching vibration bands of —CH3 and —CH2— at 2929.87 and 2875.86 cm-1, respectively, C=C at 1575.84 cm-1, vibration bands of —C—O— at 1107.14 and 1035.77 cm-1.
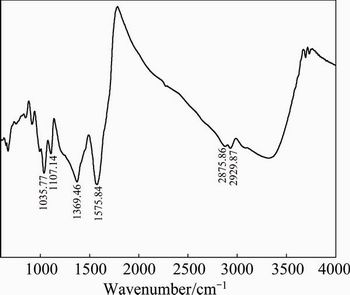
Fig. 10 Infrared spectrum of HA
Figure 11 shows the spectra obtained from galena before (Fig. 11(a)) and after (Fig. 11(b)) being oxidized by APS. The characteristic bands at 1168.86 to 1039.63 cm-1 were observed when galena was conditioned with APS, suggesting the oxidation product on galena surface was PbSO4, and the following reaction might be happened.
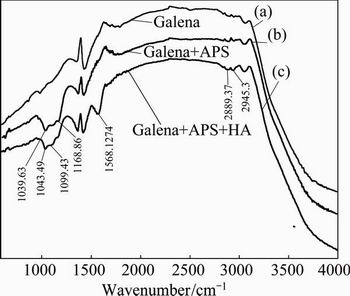
Fig. 11 Infrared spectra of galena (a), galena after being oxidized by APS (b) and HA conditioned with APS oxidized galena (c)
PbS+4S2O82-+4H2O=PbSO4+8HSO4- (1)
The spectra for the oxidized galena conditioned with HA were shown in Fig. 11(c). For the oxidized galena, characteristic bands of HA at 2929.87, 2875.86, 1575.84, 1107.14 and 1035.77 cm-1 were observed, suggesting the adsorption of HA on galena. The bands at 1575.84, 1107.14 and 1035.77 cm-1 turned to 1568.86, 1099.43 and 1043.49 cm-1, respectively, which indicated that sodium humate was chemically adsorbed on the surface of galena as the following chemical equation:
nPbSO4+2HAn-=Pbn(HA)2+nSO42- (2)
4 Conclusions
1) The mixed depressant HA+APS is highly selective in the separation of chalcopyrite and galena compared with single depressant (HA or APS). The proper oxidation of galena surface is a prerequisite for the depression of galena by HA.
2) The closed-circuit flotation test of copper/lead bulk concentrate showed that grade and recovery of Cu in copper concentrate are 30.47% and 89.16%, those of Pb are 2.06% and 1.58%, respectively; the grade and recovery of Pb in lead concentrate are 50.34% and 98.42%, those of Cu are 1.45% and 10.84%, respectively, using sodium humate (HA) and sodium persulfate (APS) as the depressants.
3) The adsorption measurement and infrared spectroscopic analysis indicated that the slurry oxidation environment and the proper oxidation of galena surface are prerequisites for the adsorption of HA on galena. HA can be hardly adsorbed on the fresh surface of galena, while after oxidation, it can be chemically adsorbed on the surface of galena.
4) The combination of HA and APS can be used as an efficient non-toxic reagent for the depressant of galena in cleaning separation for copper/lead bulk concentrate by flotation.
References
[1] LIU Jian, WEN Shu-ming, DENG Jiu-shuai, CHEN Xiu-min, FENG Qi-cheng. DFT study of ethyl xanthate interaction with sphalerite (110) surface in the absence and presence of copper [J]. Applied Surface Science, 2014, 311: 258-263.
[2] WILLS B A. Wills’ mineral processing technology: An introduction to the practical aspects of ore treatment and mineral recovery [M]. Oxford: Butterworth-Heinemann, 2011: 336-343.
[3] BULATOVIC S M. Handbook of flotation reagents: Chemistry, theory and practice [M]. Amsterdam: Elsevier, 2007: 383-389.
[4] PIAO Zheng-jie, WEI De-zhou, LIU Zhi-lin, LIU Wen-gang, GAO Shu-ling, LI Ming-yang. Selective depression of galena and chalcopyrite by O,O-bis(2,3-dihydroxypropyl) dithiophosphate [J]. Transactions of Nonferrous Metals Society of China, 2013, 23(10): 3063-3067.
[5] LI Qian, JIANG Tao, YANG Yong-bing, LI Guang-hui, GUO Yu-feng, QIU Guan-zhou. Co-intensification of cyanide leaching gold by mercury ions and oxidant [J]. Transactions of Nonferrous Metals Society of China, 2010, 20(8): 1521-1526.
[6] LIU Q, LASKOWSKI J S. The role of metal hydroxides at mineral surfaces in dextrin adsorption, II. Chalcopyrite-galena separations in the presence of dextrin [J]. International Journal of Mineral Processing, 1989, 27(1-2): 147-155.
[7] DRZYMALA J, KAPUSNIAK J, TOMASIK P. Removal of lead minerals from copper industrial flotation concentrates by xanthate flotation in the presence of dextrin [J]. International Journal of Mineral Processing, 2003, 70(1-4): 147-155.
[8] HUANG Hong-jun, HU Zhi-kai, SUN Wei, DONG Yan-hong. Research on mechanism and effects of a new inhibitor on the separation of chalcopyrite and galena [J]. Multipurpose Utilization of Mineral Resources, 2011, 172(6): 44-48. (in Chinese)
[9] LIU Run-qing, SUN Wei, HU Yue-hua. Study on organic depressant FCLS for separation of chalcopyrite and galena [J]. Mining and Metallurgical Engineering, 2009, 29(3): 29-32. (in Chinese)
[10] CHEN Jian-hua, LI Yu-qiong, CHEN Ye. Cu–S flotation separation via the combination of sodium humate and lime in a low pH medium [J]. Minerals Engineering, 2011, 24(1): 58-63.
[11] QIU Ting-sheng, ZHANG Bao-hong, ZHANG Wei-xing, ZHAO Guan-fei. Current status and progress of technology of separating arsenopyrite and pyrite [J]. Mining & Processing Equipment, 2013, 41(4): 1-5. (in Chinese)
[12] LI Xing-hai, LI Chang-cheng, YANG Wen, HUANG Wen-zheng, ZHU Fang-zhao, HE Zhi-quan, HU Xi-zheng. The separation flotation of Cu/Pb and S/As minerals by sodium (ammonium) humate [J]. Nonferrous Metals: (Mineral Processing Section), 1988, 4(4): 11-14. (in Chinese)
[13] LIANG Jing-dong, QIU Liang-bang, HU Qing-yong, ZOU Yu-zhi. Studies on separation of the bulk concentrates of Cu and Pb with sodium humate [J]. Mining and Metallurgical Engineering, 1985, 5(2): 21-24. (in Chinese)
[14] SOMASUNDARAN P, WANG D. Developments in mineral processing [M]. Amsterdam: Elsevier, 2006: 1-4.
[15] ZHUANG Guo-shun, LIAO Wen-zhuo, PAN Jie-zai, CHEN Song. Apparent stability constant of Cu- and Pb-humic acid complexes from Changjiang estuary suface sediment [J]. Jouranl of Oceanocraphy in Taiwan Strait, 1988, 4(4): 51-55. (in Chinese)
[16] TAGGART A F, BEHRE H A, BREERWOOD C H. Handbook of mineral dressing: ores and industrial minerals [M]. New York: Wiley, 1945.
[17] YIKAI K, HUIRU D. Handbook of analytical chemistry (Volume 3) [M]. 2nd ed. Beijing: Chemical Industry Press, 2004: 928-980.
腐殖酸钠和过硫酸铵组合抑制剂浮选分离黄铜矿和方铅矿
刘瑞增1,覃文庆1,焦 芬1,王兴杰1,裴 斌2,杨永军2,赖春华3
1. 中南大学 资源加工与生物工程学院,长沙 410083;
2. 巴润矿业公司,内蒙古包钢钢联股份有限公司,包头 014080;
3. 正源矿业有限责任公司,红河 662400
摘 要:采用浮选实验、吸附量测量以及红外光谱分析等方法研究腐殖酸钠和过硫酸铵对黄铜矿和方铅矿浮选行为的影响和机理。单矿物浮选实验表明,表面适当氧化是方铅矿受到腐蚀酸钠抑制的先决条件。以腐殖酸钠和过硫酸铵为方铅矿抑制剂的铜铅混合精矿闭路实验可以得到铜品位和回收率分别为30.47%和89.16%,铅品位和回收率分别为2.06%和1.58%的铜精矿,以及铅品位和回收率分别为50.34% 和98.42%,铜品位和回收率分别为1.45% 和10.84%的铅精矿的抑制效果优于重铬酸钾。吸附量和红外光谱测试表明,腐殖酸钠虽然基本不会在新鲜的方铅矿表面发生吸附,但是能大量吸附在适当氧化后的方铅矿表面。根据溶度积理论,方铅矿表面氧化后生成PbSO4,腐殖酸钠与其发生置换反应后能以化学吸附的方式吸附在氧化后的方铅矿表面,从而产生抑制效果。腐殖酸钠和过硫酸铵组合抑制剂是铜铅混合精矿清洁分离的有效方法。
关键词:黄铜矿;方铅矿;腐殖酸钠;过硫酸铵;浮选
(Edited by Yun-bin HE)
Foundation item: Project (51274255) supported by the National Natural Science Foundation of China; Project supported by the Co-innovation Center for Clean and Efficient Utilization of Strategic Metal Mineral Resources, China
Corresponding author: Wen-qing QIN; Tel: +86-731-88830884; E-mail: qinwenqing369@126.com
DOI: 10.1016/S1003-6326(16)64113-4