
Coarsening rate of microstructure in semi-solid aluminium alloys
H. V. ATKINSON1, D. LIU1, 2
1. Mechanics of Materials Research Group, Department of Engineering, University of Leicester,
University Rd., Leicester, LE1 7RH, UK;
2. Now with Wolfson Microelectronics PLC., 26, Westfield Rd., Edinburgh, EH11 2QB, UK
Received 13 May 2010; accepted 25 June 2010
Abstract: The rate of microstructural coarsening of recrystallisation and partial melting (RAP) and cooling slope (CS) processed materials in the semi-solid state is compared with rates found in the literature. The rate of coarsening depended on the liquid fraction but RAP route 2014 alloy with 37% liquid coarsened slightly more slowly than the CS route 2014 alloy with a lower liquid fraction of 17%, contrary to expectations. For the CS route, an increase in liquid fraction resulted in faster coarsening. A modified 2014 alloy with Fe, Mn and Zn stripped out of the composition gave a relatively high coarsening rate. The coarsening rate was also relatively high for CS 201 alloy in comparison with either RAP 2014 or CS 2014. Low coarsening rates are thought to be associated with the presence of particles which inhibit the migration of liquid film grain boundaries. This could be the result of pinning or of the liquid film impeding diffusion at the boundary.
Key words: coarsening; semi-solid metal; 2014 alloy; recrystallisation and partial melting; cooling slope
1 Introduction
Semi-solid processing takes place between the liquidus and solidus temperatures and requires the microstructure in the semi-solid state to consist of spheroids of solid in a liquid matrix[1]. The material then behaves thixotropically, i.e. it flows when being sheared but thickens when being allowed to stand. Hence, processing routes based on this behaviour are termed thixocasting (when the liquid fraction is relatively high) and thixoforging (when the liquid fraction is relatively low). The term thixoforming is either used to denote a intermediate process between these two in terms of liquid fraction or as a general description for the semi-solid processing routes which involve reheating solid into the semi-solid state. During reheating, the microstructure evolves; extensive coarsening will have a deleterious effect on the final mechanical properties of the thixoformed component. As a rule, if the spheroid size on forming is greater than about 100 μm, the properties are likely to be unacceptable. In addition, if the spheroid size is too large, it will limit flow into thin sections of the die (with a 1 mm-thick section equivalent to 10 spheroid diameters if each spheroid is 100 μm).
In this work, the rates of coarsening in material produced by two different routes: recrystallisation and partial melting (RAP)[2] and cooling slope casting (CS)[3], are compared. RAP is used for the commercial production of aluminium alloy components. CS is associated with the new rheocasting route (NRC)[4-5] and also has wider potential applications[6-7] although there is the potential for incorporation of oxide. RAP involves material which has been previously worked, e.g. by extrusion or rolling. When this material is reheated into the semi-solid state, it recrystallizes and the first liquid to form tends to be on the recrystallized boundaries. RAP is distinct from SIMA (strain induced melt activation) process[8] in that the working is ‘warm’ whereas in SIMA it is ‘hot’. Many aerospace alloys are supplied in the worked state and therefore are immediately suitable for reheating and thixoforming. In addition, the spheroids tend to be very well rounded in comparison with those produced by liquid state routes such as magnetohydrodynamic (MHD) stirring, tending to flow better[9].
In the cooling slope route, liquid metal is poured onto a chilled slope and as it runs down, solid nuclei develop. These tumble over each other in the flow, preventing dendrite development and hence producing a solid material which is suitable for reheating for thixoforming[10].
Classically, for low volume fractions of solid φs, the LSW theory[11-13] is applied:
(1)
where
is the average particle diameter at time t,
is the average particle diameter at time t=0 and K is the coarsening rate constant. For higher φs, ANNAVARAPU and DOHERTY[14] proposed the liquid film migration model where the coarsening rate should increase as φs increases. However, experimental results[15] show the opposite. A modified liquid film migration model was therefore developed by MANSON-WHITTON et al[15] to take into account of the necks between the contacting solid particles. The transition between the two occurs at φs about 0.7. Other works[16-17] have observed that at high φs the coarsening is controlled by diffusion through the liquid rather than by solid-solid contacts. MANSON-WHITTON et al[15] and de FREITAS et al[18] have discussed the inhibition of coarsening by particles present in grain boundary liquid films.
In this work, aluminium alloy material produced by this route and by RAP is analysed and compared with results in the literature. A longer, more detailed version has previously been presented[19]. The materials are RAP 2014 (a conventionally wrought alloy); CS 2014, a modified 2014 without the minor elements Fe, Mn and Zn and with relatively low Si produced by CS; and alloy 201 (a high strength casting alloy) produced by CS. The alloys have previously been thixoformed and the results have been presented[20-21].
2 Experimental
The condition of starting materials is summarized in Table 1. The extruded 2014 was used for both the RAP route and for remelting for the CS. The details for the cooling slope route are given in Ref.[19]. The modified 2014 and 201 were both treated with the CS. A salt bath furnace was used to study the microstructural evolution in the semi-solid state. The temperatures were originally chosen to give fractions of liquid suitable or approaching those suitable for thixoforming of the alloys concerned. It is, therefore, not the case that the same liquid fraction has been used for each alloy. The samples were 8 mm×8 mm×15 mm in size and were monitored with a thermocouple in a hole in the centre of the specimen. The isothermal holding temperatures are shown in Table 2 along with the liquid volume fraction determined with differential scanning calorimetry (DSC). After heat treatment, the samples were ground, polished and examined optically. Image analysis was used to obtain the grain/rosette size (d=(4A/π)1/2, where A is area of rosette) and the coarsening rate constants calculated using Eq.(1). The term ‘rosette’ is used here to describe the microstructural features which are not dendrites but are not fully rounded spheroids either.
Table 1 Compositions of starting materials with wrought and cast handbook values shown for comparison (mass fraction, %)
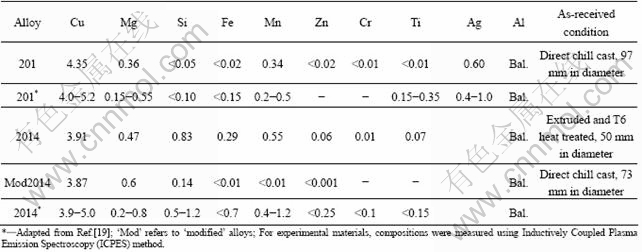
Table 2 Temperatures of isothermal holding and corresponding liquid fractions (adapted from Ref.[19])
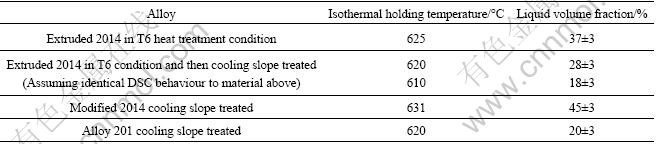
Micrograph sequences for isothermal holding in the semi-solid state have been previously presented for extruded 2014 (T6 condition), cooling slope modified 2014 and cooling slope 201[20]. The results for cooling slope 2014 are shown in Ref.[19]. We focus here on results for the coarsening rate constants.
3 Results and discussion
Fig.1 shows the results for the evolution of the cube of the average globule diameter as a function of the isothermal soaking time in the semi-solid state for the various alloys in Table 1. The intercept is the value of
. As commented previously, the amounts of liquid are not directly comparable. However, it is clear that the alloy 2014, made by either the CS or the RAP route gave slower coarsening kinetics. The values of the coarsening rate constant are shown in the graph. A lower coarsening rate constant was observed for the RAP route than for the CS route and a lower coarsening rate for the CS route at lower heating temperature with lower fraction liquid. Compared with alloy 2014, relatively faster coarsening kinetics were shown by modified alloy 2014 and alloy 201 made by CS.
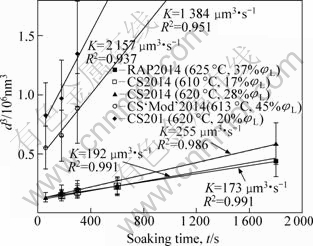
Fig.1 Evolution of cube of average globule diameter as function of isothermal soaking time at 610-631 °C in semi-solid state for different materials (adapted from Ref.[19])
Coarsening rate thus appears to be related to the initial microstructure, i.e. RAP or CS, and to the alloy composition. For example, for a similar initial microstructure from cooling slope casting, a higher coarsening rate constant was obtained for modified 2014 and alloy 201 than for unmodified 2014.
Some liquid pockets are present within the primary phase. An example is shown in Fig.2.
During partial melting and then holding in the semi-solid state, coarsening first proceeds through the coalescence of dendrite arms (Fig.2). After coalescence, phase coarsening will take place through the dissolution of the small globules and the grain numbers will then decrease[22]. In the alloys examined here, micrographs show that coalescence has a relatively minor effect after the very initial stages. CS 2014, CS cast modified 2014 and alloy 201 all have similar Cu contents. The differences in coarsening rates are thought to be due to secondary phases[15-18]. The precipitation hardening particles are not responsible because they tend to dissolve below the solidus. It is more likely to be the Fe, Mn-based intermetallic particles, which are insoluble, giving the pinning effect. Hence, the modified alloy with these elements stripped out coarsens fast. This could be the result of pinning or of the liquid film impeding diffusion at the boundary. It is likely that the difference between RAP 2014 and CS 2014 is due to differences between quantities and distributions of the intermetallic particles.
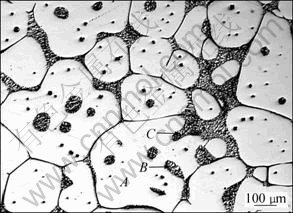
Fig.2 Presence of entrapped liquid in primary grains in cooling slope modified alloy 2014 soaked for 5 min at 631 °C (adapted from Ref.[19])
Figs.3 and 4 summarise data from the literature. The majority of the results in Fig.4 are for wrought alloys which are likely to have minority phase particles present.
The exceptions are circled. The AlCu4.5Mg1.5[14] results are for a grain refined alloy and it may be the Ti-rich grain refining particles which inhibit grain boundary migration in the semi-solid state. There are also some results[24] which may be explained by the presence of porosity in small sprayforming batches[31].
4 Conclusions
Two potential routes to suitable material for thixoforming are RAP and CS. Here the microstructural coarsening of such materials in the semi-solid state has been analysed. A lower coarsening rate was observed for RAP route 2014 than for CS route 2014 despite the higher liquid fraction for the former. For the CS route, an increase in fraction liquid gave a higher coarsening rate. A relatively high coarsening rate was observed for ‘modified’ 2014 with the Fe, Mn and Zn not present in the composition and also for CS 201. Dendrite arm coalescence only contributes to coarsening in the very initial stages with CS material. The lower coarsening rate in CS 2014 in comparison with other alloys is thought to be due to the presence of secondary phases in the 2014 which pin the liquid boundary migration. The particles must be resistant to dissolution and are likely to be insoluble Fe,Mn-based intermetallics. Analysis of data from the literature suggests that those alloys which give relatively low coarsening rate coefficients generally have pinned liquid film boundaries (e.g. due to intermetallics, porosity, or grain refining species such as Ti). Thus, wrought alloy compositions tend to coarsen more slowly than cast one, as alloys do which contain grain refiner.
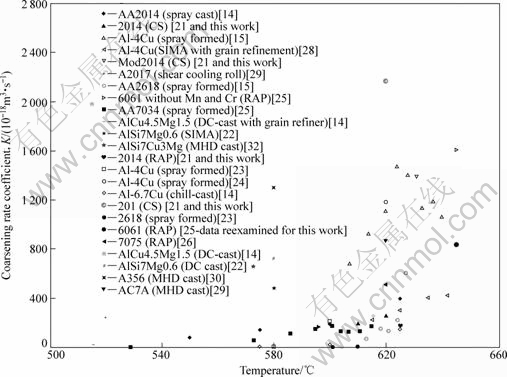
Fig.3 Coarsening rate coefficient (K) vs temperature assuming d3-d03=Kt for Al alloys showing generally high K (>600 μm3/s) in casting Al alloys and generally low K (<600 μm3/s) in normally wrought alloys (adapted from Ref.[19])
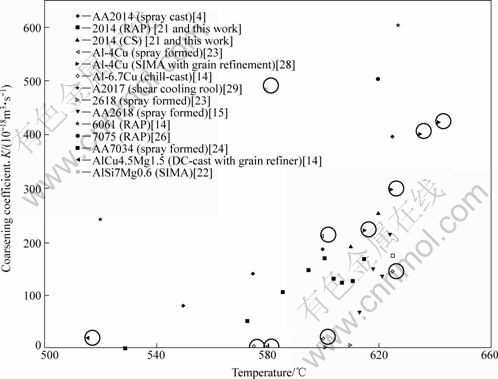
Fig.4 Magnified view of (0-650)×10-18 m3/s range in K in Fig.3 (adapted from Ref.[19])
Acknowledgements
The authors would like to thank the UK Engineering and Physical Sciences Research Council (EPSRC Grant GR/M89096) and the University of Leicester for financial support. The University of Sheffield provided experimental facilities.
References
[1] FLEMINGS M C. Behaviour of metal alloys in the semisolid state [J]. Metall Trans A, 1991, 22: 957-981.
[2] KIRKWOOD D H, SELLARS C M, ELIAS BOYED L G. Thixotropic materials. European Patent 0305375 B1 [P]. 1992-10-28.
[3] HAGA T, KOUDA H, MOTOYAMA N, INOUE N, SUZUKI S. High speed roll caster for aluminium alloy strip [C]// Proceedings of ICAA7, Aluminium Alloys: Their Physical and Mechanical Properties. Charlottesville, VA: Trans Tech Publications, 2000: 327-332.
[4] KANEUCHI T, SHIBATA R, OZAWA M. Development of a new semi-solid metal casting process for automotive suspension parts [C]// Proc 7th Int Conf on Advanced Semi-Solid Processing of Alloys and Composites. TSUTSUI Y, KIUCHI M, ICHIKAWA K. Tsukuba, Japan: National Institute of Advanced Science and Technology and the Japan Society for Technology of Plasticity, 2002: 145-150.
[5] MITSURU A, HIROTO S, YASUNORI H, TATSUO S, SATORU S, ATSUSHI Y. Method and Apparatus for Shaping Semisolid metals. Patent EP 0745694 A1 [P]. 1996.
[6] HAGA T. Semisolid strip casting using a twin roll caster [J]. J Mater Process Technol, 2002, 130/131: 558-561.
[7] LIMA FILHO A D, YAMASAKI M I. Evaluation of strip rolling directly from the semisolid state [J]. Solid State Phenomena, 2006, 116/117: 433-436.
[8] YOUNG K P, KYONKA C P, COURTOIS J A. Fine Grained metal composition. US Patent 4415374 [P]. 1982-03-30.
[9] LIU T Y, ATKINSON H V, KAPRANOS P, KIRKWOOD D H, HOGG S C. Rapid compression of aluminium alloys [J]. Metall Mater Trans A, 2003, 34: 1545-1554.
[10] CARDOSA LEGORETTA E, ATKINSON H V, JONES H. Cooling slope casting to obtain thixotropic feedstock II: Observations with A356 alloy [J]. J Mater Sci, 2008, 43: 5456-5469.
[11] GREENWOOD G W. The growth of dispersed precipitates in solutions [J]. Acta Metall, 1956, 4: 253-248.
[12] LIFSHITZ I M, SLYOZOV V V. The kinetics of precipitation from supersaturated solid solutions [J]. J Phys Chem Solids, 1961, 19: 35-50.
[13] WAGNER C. Theorie der altering von niederschl?gen durch uml?sen (ostwald-reifung) [J]. Z. Electrochemie, 1961, 65: 581-591.
[14] ANNAVARAPU S, DOHERTY R D. Inhibited coarsening of solid-liquid microstructures in spray casting at high volume fractions of solid [J]. Acta Metall Mater, 1995, 43: 3207-3230.
[15] MANSON-WHITTON D, STONE I C, JONES J R, GRANT P S, CANTOR B. Isothermal grain coarsening of spray formed alloys in the semisolid state [J]. Acta Mater, 2002, 50: 2517-2535.
[16] KIM S S, YOON D N. Coarsening of Mo grains dispersed in liquid matrix [J]. Acta Metall, 1983, 31: 1151-1157.
[17] BOETTINGER W J, VOORHEES P W, DOBBYN R C, BURDETTE H E. A study of the coarsening of liquid-solid mixtures using synchrotron radiation microradiography [J] Metall Trans A, 1987, 18: 487-490.
[18] de FREITAS E R, FERRACINI E Jr, FERRANTE M. Microstructure and rheology of an AA2024 aluminium alloy in the semisolid state, and mechanical properties of a back-extruded part [J]. J Mater Process Technol, 2004, 146: 241-249.
[19] ATKINSON H V, LIU Dan. Microstructural coarsening of semi-solid aluminium alloys [J]. Mater Sci Eng A, 2008, 496: 439-446.
[20] LIU Dan, ATKINSON H V, KAPRANOS P, JIRATTITICHAROEAN W, JONES H. Microstructural evolution and tensile mechanical properties of thixoformed high performance aluminium alloys [J]. Mater Sci Eng A, 2003, 361: 213-224.
[21] LIU Dan. Thixoforming of high performance alloys mainly based on the Al-Cu system [D]. Sheffield: University of Sheffield, 2003.
[22] LOUE W R, Suery M. Microstructural evolution during partial remelting [J]. Mater Sci Eng A, 1995, 203: 1-13.
[23] GRANT P S, UNDERHILL R P, KIM W T, MINGARD K P, CANTOR B. [C]//WOOD J V. Spray Forming 2. Woodhead, Cambridge, 1993: 45.
[24] KIM Hee-Soo. Microstructure and deformation behavior of semi-solid aluminium alloys [D]. Oxford: University of Oxford, 2003.
[25] PITTS H. Semi-solid processing of aluminium 6061 [D]. Sheffield: University of Sheffield, 1999: 52.
[26] CHAYONG S. Thixoforming processing of aluminium 7075 alloy [D]. Sheffield: University of Sheffield, 2002: 98.
[27] SALVO L, SUERY M, CHARENTENAY Y, LOUE W. Microstructural evolution and rheological behaviour in the semi-solid state of a new Al-Si based alloy [C]//Proc 4th Int Conf Semi-Solid Processing of Alloys and Composites. Sheffield, UK, 1996: 10-15.
[28] FERRANTE M, de FREITAS E. Rheology and microstructural development of a Al-4wt% Cu alloy in the semi-solid state [J]. Mater Sci Eng A, 1999, 271: 172-180.
[29] KIUCHI M, YANAGIMOTO J, SUGIYAMA S. Discussion on microstructures of mushy alloys in heating process [C]//Proc 5th Int Conf on Semi-Solid Processing of Alloys and Composites, Colorado, USA, 1998: 589-596.
[30] ZOQUI E J, SHEHATA M T, PAES M, KAO V, ES-SADIQI E. Morphological evolution of SSM A356 during partial remelting [J]. Mater Sci Eng A, 2002, 325: 38-53.
[31] GRANT P S. Oxford University, Private Communication, Jan 2008.
(Edited by YANG Bing)
Corresponding author: H. V. ATKINSON; Tel: +44-116-2231019; E-mail: hva2@le.ac.uk
DOI: 10.1016/S1003-6326(09)60356-3